Dibenzo-30-crown-10
CAS No.: 17455-25-3
Cat. No.: VC21058827
Molecular Formula: C28H40O10
Molecular Weight: 536.6 g/mol
* For research use only. Not for human or veterinary use.
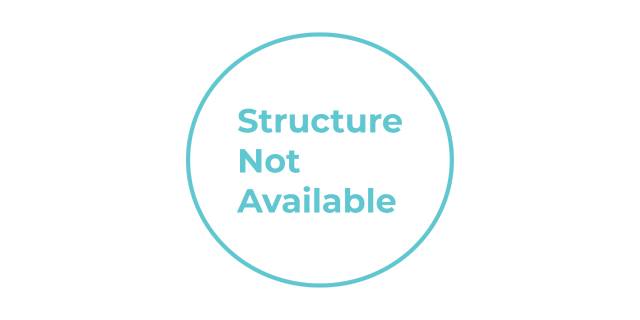
Specification
CAS No. | 17455-25-3 |
---|---|
Molecular Formula | C28H40O10 |
Molecular Weight | 536.6 g/mol |
IUPAC Name | 2,5,8,11,14,21,24,27,30,33-decaoxatricyclo[32.4.0.015,20]octatriaconta-1(38),15,17,19,34,36-hexaene |
Standard InChI | InChI=1S/C28H40O10/c1-2-6-26-25(5-1)35-21-17-31-13-9-29-11-15-33-19-23-37-27-7-3-4-8-28(27)38-24-20-34-16-12-30-10-14-32-18-22-36-26/h1-8H,9-24H2 |
Standard InChI Key | MXCSCGGRLMRZMF-UHFFFAOYSA-N |
SMILES | C1COCCOC2=CC=CC=C2OCCOCCOCCOCCOC3=CC=CC=C3OCCOCCO1 |
Canonical SMILES | C1COCCOC2=CC=CC=C2OCCOCCOCCOCCOC3=CC=CC=C3OCCOCCO1 |
Introduction
Chemical Structure and Physical Properties
Dibenzo-30-crown-10, systematically named dibenzo-1,4,7,10,13,16,19,22,25,28-decaoxacyclotriacontane, is characterized by its large 30-membered ring containing 10 oxygen atoms and two benzene rings incorporated into the macrocyclic skeleton. The compound has a molecular formula of C₂₈H₄₀O₁₀ and a molecular weight of 536.62 g/mol . Its structure features dual benzoate and ether group composition, which contributes to its unique binding properties through hydrogen bonding interactions between the benzoate and ether groups .
Key Physical Properties
Property | Value |
---|---|
Chemical Formula | C₂₈H₄₀O₁₀ |
Molecular Weight | 536.62 g/mol |
Melting Point | 106-108°C |
Physical Appearance | Crystalline solid |
Systematic Name | Dibenzo-1,4,7,10,13,16,19,22,25,28-decaoxacyclotriacontane |
The unique arrangement of oxygen atoms in the crown ether ring creates a cavity that can selectively bind to various cations and organic molecules, making Dibenzo-30-crown-10 a versatile host molecule in supramolecular chemistry. The benzene rings provide rigidity to certain segments of the macrocycle while still allowing considerable conformational flexibility .
Historical Context and Discovery
Dibenzo-30-crown-10 is one of the first-generation macrocyclic hosts discovered by Charles J. Pedersen, who first reported crown ethers in 1967 . Crown ethers initially attracted attention due to their remarkable ability to encapsulate metal cations and render them soluble in organic solvents, a property that helped launch host-guest chemistry as a discipline within supramolecular chemistry .
Despite being reported in 1967 and commercially available since 1992, Dibenzo-30-crown-10 has been surprisingly overlooked in favor of smaller crown ethers such as DB24C8, B15C5, 18C6, and 15C5 . This historical neglect is particularly puzzling given its exceptional binding properties and potential applications. Only recently has there been a resurgence of interest in this larger crown ether as researchers recognize its unique capabilities in various chemical and industrial processes.
Synthesis and Optimization
The traditional synthesis of Dibenzo-30-crown-10 faced significant limitations, particularly in the cyclization step which historically achieved yields of only approximately 25% . Recent advancements in synthetic methodology have dramatically improved this process.
Synthetic Improvements
Researchers have developed an improved synthetic route that increases the yield of the cyclization step from 25% to an impressive 88% . This optimization enables the preparation of multiple grams of Dibenzo-30-crown-10 without the use of pseudo high-dilution techniques, which were previously necessary for macrocycle formation .
Synthetic Approach | Cyclization Step Yield |
---|---|
Traditional Method | 25% |
Improved Method | 88% |
The same improved methodology has been successfully applied to three other crown ethers with similar yield improvements, demonstrating the broader applicability of this synthetic approach . This significant enhancement in synthetic efficiency makes Dibenzo-30-crown-10 more accessible for research and potential industrial applications.
Complexation Behavior
Metal Cation Complexes
Dibenzo-30-crown-10 exhibits exceptional complexation properties with metal cations, particularly with potassium and rare earth elements. Crystal structure analyses have provided detailed information about these complexes. In the potassium complex ([K(DB30C10)]⁺PF₆⁻), the potassium atom is coordinated to all ten oxygen atoms of the crown ligand at distances ranging from 2.859(3) to 2.930(3) Å . This coordination geometry demonstrates the ability of the large crown cavity to completely encapsulate the potassium ion in a stable configuration.
Recent studies have highlighted Dibenzo-30-crown-10 as one of the most efficient complexants for the separation of rare earth mixtures based on cation size . Molecular dynamics simulations have provided insights into the interactions between Dibenzo-30-crown-10 and rare earth elements like samarium (Sm²⁺) and europium (Eu²⁺) . These simulations revealed that the Gibbs binding free energies of Dibenzo-30-crown-10 with SmBr₂ and EuBr₂ are nearly identical, with Sm²⁺ being slightly more favorable .
Organic Cation Complexes
Beyond metal cations, Dibenzo-30-crown-10 also forms stable complexes with organic cations such as ammonium. In the ammonium complex ([NH₄(DB30C10)]⁺PF₆⁻), the ammonium cation is completely enclosed by the crown oxygen atoms, forming seven hydrogen bonds . The crystal structure analysis showed that this complex maintains a 1:1 crown-cation ratio, similar to the potassium complex .
Complex | Space Group | Unit Cell Parameters | Density (g/cm³) |
---|---|---|---|
[K(DB30C10)]⁺PF₆⁻ | P2/n | a = 11.9106(3) Å, b = 9.8382(5) Å, c = 14.3062(3) Å, β = 97.581(3)° | 1.440 |
[NH₄(DB30C10)]⁺PF₆⁻ | P21/n | a = 12.5061(6) Å, b = 19.3724(5) Å, c = 14.2203(9) Å, β = 102.476(5)° | 1.501 |
The extensive hydrogen bonding network contributes significantly to the stability of the ammonium complex, highlighting the versatility of Dibenzo-30-crown-10 as a host molecule for different types of guests .
Viologen and Diquat Complexation
A particularly fascinating aspect of Dibenzo-30-crown-10's complexation behavior is its interaction with viologens (4,4'-bipyridinium derivatives) and diquat dication. Recent research has demonstrated for the first time that Dibenzo-30-crown-10 forms pseudorotaxane complexes with viologens in solution . Several rotaxanes based on the Dibenzo-30-crown-10/viologen binding motif have been synthesized and characterized using ¹H NMR, ¹³C NMR, and HRMS .
An X-ray crystal structure of one of these rotaxanes has been obtained, providing valuable insights into the structural features of these complexes . This represents a significant advancement in understanding the binding interactions between Dibenzo-30-crown-10 and viologen derivatives.
Additionally, investigations into the complex between the diquat dication and Dibenzo-30-crown-10 revealed that weak C-H⋯O hydrogen bonding and charge transfer are the major components of host-guest binding . These same components are responsible for the formation of stable and ordered 1:1 solution complexes between dibenzo-3n-crown-n (n = 9-12) and diquat bis(hexafluorophosphate) .
Conformational Studies
Conformational analysis of Dibenzo-30-crown-10 and its complexes has provided crucial insights into the flexibility and adaptability of this macrocycle. Polar coordinate maps have been used to investigate the conformation of Dibenzo-30-crown-10 complexes with potassium salts . These studies have revealed the dynamic nature of the crown ether ring and how it adapts to accommodate different guest molecules.
Molecular dynamics simulations have uncovered fascinating conformational behaviors in Dibenzo-30-crown-10 systems. Large conformational fluctuations have been observed to be dependent on both the identity of the lanthanide and halide complexes . For instance, in systems with chloride and bromide anions, no conformational changes were observed over a 200 ns simulation period, while in iodide systems, there were two conformational changes with Sm²⁺ and one with Eu²⁺ within the same timeframe .
Particularly noteworthy is the conformational transition observed in the SmI₂-Dibenzo-30-crown-10 system, which exhibited three distinct stages of conformational changes :
-
Initial unfolded conformation
-
Partly folded intermediate state
-
Final completely folded conformation
This folding mechanism provides valuable insights into the adaptability of Dibenzo-30-crown-10 when interacting with different guest species and highlights the complex conformational landscape of this flexible macrocycle.
Applications
Rare Earth Separation
One of the most promising applications of Dibenzo-30-crown-10 is in the separation of rare earth elements. It has been identified as one of the most efficient complexants for the separation of rare earth mixtures based on cation size . This property is particularly valuable in industrial applications related to rare earth mining and refining.
The ability of Dibenzo-30-crown-10 to discriminate between closely related lanthanides makes it a powerful tool for selective extraction and purification processes. Calculations comparing the Gibbs binding free energies of Dibenzo-30-crown-10 and dicyclohexano-18-crown-6 (DCH18C6) with SmI₂ have shown that Dibenzo-30-crown-10 exhibits more favorable complexation affinities , further supporting its potential in rare earth separation applications.
Supramolecular Chemistry
Dibenzo-30-crown-10 has proven to be a valuable building block in supramolecular chemistry, particularly in the construction of mechanically interlocked molecules such as rotaxanes and catenanes. The successful synthesis of rotaxanes based on the Dibenzo-30-crown-10/viologen binding motif has demonstrated the suitability of this crown ether for constructing complex supramolecular architectures .
These structures have potential applications in the development of molecular machines, switches, and stimuli-responsive materials. Additionally, Dibenzo-30-crown-10 has been used in the construction of supramolecular polymers with novel topologies , opening up new possibilities in materials science and nanotechnology.
Environmental Applications
The unique binding properties of Dibenzo-30-crown-10 make it useful for environmental applications, particularly in wastewater treatment. It has shown efficiency as an adsorbent for the removal of lysine residues from wastewater . The selective binding capabilities of crown ethers like Dibenzo-30-crown-10 can be harnessed for the targeted removal of specific contaminants from water sources, offering potential solutions for challenging environmental remediation problems.
Analytical Chemistry
Recent Research Developments
Recent years have seen a resurgence of interest in Dibenzo-30-crown-10, with new studies exploring its properties and potential applications using advanced computational and experimental techniques. Molecular dynamics simulations using the polarizable atomic multipole optimized energetics for biomolecular simulation (AMOEBA) force field have provided detailed insights into the interactions between Dibenzo-30-crown-10 and rare earth elements .
These simulations have revealed the complex conformational behavior of Dibenzo-30-crown-10 in different chemical environments and have helped elucidate the factors that influence its binding selectivity. For example, the large conformational fluctuations present in Dibenzo-30-crown-10 systems were found to be dependent on both the identity of the lanthanide and halide complexes .
Another significant development is the first crystal structure of a rotaxane based on the Dibenzo-30-crown-10-paraquat binding motif , providing valuable structural information that can guide the design of new supramolecular systems based on this binding motif. Additionally, studies on the threading efficiency of Dibenzo-30-crown-10 in solution have provided insights into its behavior in the construction of supramolecular polymers .
- mass of a compound required to prepare a solution of known volume and concentration
- volume of solution required to dissolve a compound of known mass to a desired concentration
- concentration of a solution resulting from a known mass of compound in a specific volume