N-Benzylidenebenzylamine
CAS No.: 780-25-6
Cat. No.: VC1965282
Molecular Formula: C14H13N
Molecular Weight: 195.26 g/mol
* For research use only. Not for human or veterinary use.
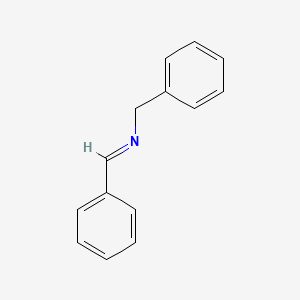
Specification
CAS No. | 780-25-6 |
---|---|
Molecular Formula | C14H13N |
Molecular Weight | 195.26 g/mol |
IUPAC Name | N-benzyl-1-phenylmethanimine |
Standard InChI | InChI=1S/C14H13N/c1-3-7-13(8-4-1)11-15-12-14-9-5-2-6-10-14/h1-11H,12H2 |
Standard InChI Key | MIYKHJXFICMPOJ-UHFFFAOYSA-N |
SMILES | C1=CC=C(C=C1)CN=CC2=CC=CC=C2 |
Canonical SMILES | C1=CC=C(C=C1)CN=CC2=CC=CC=C2 |
Introduction
Chemical Structure and Properties
N-Benzylidenebenzylamine has the molecular formula C₁₄H₁₃N and is represented by the structural formula C₆H₅CH₂N=CHC₆H₅. This structure highlights the imine (C=N) bond that characterizes all Schiff bases. The compound appears as a pale-yellow oily product at room temperature .
Spectroscopic Characteristics
Spectroscopic analysis provides definitive confirmation of N-benzylidenebenzylamine's structure:
¹H-NMR Spectroscopy (400 MHz, CDCl₃):
-
δ 8.40 (s, 1H): Corresponds to the imine proton (N=CH)
-
δ 7.80–7.77 (m, 2H): Aromatic protons
-
δ 7.43–7.41 (m, 3H): Aromatic protons
-
δ 7.35–7.34 (m, 4H): Aromatic protons
-
δ 7.29–7.26 (m, 1H): Aromatic proton
¹³C-NMR Spectroscopy (400 MHz, CDCl₃):
-
δ 162 ppm: Imine carbon (C=N)
-
δ 139.23, 136.09, 130.78, 128.61, 128.49, 128.27, 127.98, 126.99 ppm: Aromatic carbons
FTIR Spectroscopy:
-
1643 cm⁻¹: Characteristic band corresponding to the C=N double bond
Physical and Chemical Properties
N-Benzylidenebenzylamine exhibits notable stability under atmospheric conditions. When comparing with precursor benzylamine, significant differences exist in chemical behavior. While benzylamine shows strong alkaline reactions even in diluted aqueous solutions (pH 11.6 at 100 g/L), N-benzylidenebenzylamine demonstrates different reactivity patterns due to the presence of the imine functional group . The compound is relatively stable under normal storage conditions, though it is typically stabilized with 100 ppm of MEHQ (methyl ether hydroquinone) for long-term storage and laboratory use .
Synthesis Methods
N-Benzylidenebenzylamine can be synthesized through various routes, each offering different advantages in terms of yield, reaction conditions, and environmental impact.
Classical Condensation Method
The traditional method involves the condensation reaction between benzylamine and benzaldehyde, typically carried out in solvents like ethanol or methanol at room temperature:
C₆H₅CH₂NH₂ + C₆H₅CHO → C₆H₅CH=NCH₂C₆H₅ + H₂O
Catalytic Oxidation Methods
Several catalytic pathways have been developed for the synthesis of N-benzylidenebenzylamine:
Iron Catalyst Method
A highly efficient method involves the interaction of benzylamine with carbon tetrachloride (CCl₄) in the presence of FeCl₃·6H₂O catalyst:
-
Temperature: 40-85°C
-
Reaction time: 0.5-8 hours
-
Molar ratio [catalyst]:[benzylamine]:[CCl₄] = 0.1-1:100:50-200
-
System: Open system
Photocatalytic Methods
Bismuth ellagate (Bi-ellagate) metal-organic framework catalyzes the conversion of benzylamine to N-benzylidenebenzylamine under visible light irradiation:
-
Conditions: Visible light irradiation for 24 hours at room temperature
-
Catalyst: Bi-ellagate (5 mg per 0.5 mmol benzylamine)
-
System: Solvent-free, open air
Metal-Based Catalytic Systems
Various metal catalysts have been employed:
-
Heteropolyoxymetallate NPV₆Mo₆ with oxygen: High selectivity in toluene
-
CuO/CeO₂-ZrO₂ catalyst system: High conversion and selectivity
-
Molybdenum oxide promoted CeO₂-SiO₂ mixed-oxide catalyst: Enhanced selectivity and yield
Comparative Analysis of Synthesis Methods
Table 1 summarizes the different synthesis methods for N-benzylidenebenzylamine:
Reaction Mechanisms
The formation of N-benzylidenebenzylamine occurs through several mechanistic pathways depending on the synthesis route employed.
Classical Condensation Mechanism
In the traditional synthesis pathway:
-
Nucleophilic attack by the nitrogen of benzylamine on the carbonyl carbon of benzaldehyde
-
Formation of a carbinolamine intermediate
-
Dehydration to form the imine (C=N) bond
Photocatalytic Oxidation Mechanism
The photocatalytic mechanism using catalysts like Bi-ellagate involves:
-
Excitation of the photocatalyst by visible light, generating electron-hole pairs
-
Benzylamine oxidation by the valence band hole to form benzylamine radical cation
-
Reaction with active - O₂⁻ radicals to form Ph-CH=NH intermediate
-
Reaction of this intermediate with another benzylamine molecule to form N-benzyl-1-phenylmethanediamine
Metal-Catalyzed Oxidation Mechanism
With metal oxide catalysts like CuO/CeO₂-ZrO₂:
-
Interaction of benzylamine and molecular oxygen with acidic and redox-active sites of the catalyst
-
Oxidation of benzylamine to form an imine intermediate
-
Reaction of the imine intermediate with another benzylamine molecule
-
Alternatively, hydrolysis of the imine intermediate to form benzaldehyde
-
Condensation of benzaldehyde with benzylamine at acidic sites to yield N-benzylidenebenzylamine
Applications
N-Benzylidenebenzylamine exhibits versatility across multiple scientific and industrial domains.
Synthetic Chemistry Applications
In organic synthesis, N-benzylidenebenzylamine serves as a valuable intermediate:
-
Synthesis of heterocycles, particularly substituted piperidin-2-ones
-
Preparation of compounds with physiological activities
-
Participates in reactions like Diels-Alder, Umpolung, and Aza-Baylis-Hillman reactions
Analytical Chemistry Applications
The compound finds various uses in analytical chemistry:
Microscopy and Imaging Applications
N-Benzylidenebenzylamine enhances microscopic imaging:
-
Increases contrast and clarity of samples in biological microscopy
-
Improves visualization capabilities in research applications
Pharmaceutical and Biological Applications
The compound shows promise in pharmaceutical applications:
-
Potential enhancer of stability and efficacy of active pharmaceutical ingredients
-
Contributes to the development of compounds with diverse biological activities
Industrial Applications
Several industrial uses have been identified:
Recent Research Developments
Research on N-benzylidenebenzylamine continues to evolve, focusing on improved synthesis methods and expanded applications.
Advanced Catalytic Systems
Recent developments in catalyst design have enhanced the efficiency of N-benzylidenebenzylamine synthesis:
Nanostructured Catalysts
Copper-supported doped-CeO₂ catalysts demonstrate improved efficiency for benzylamine oxidation:
-
Enhanced catalytic activity through rational design
-
Effective in both absence and presence of specific conditions
Mixed-Oxide Catalysts
Molybdenum oxide promoted CeO₂-SiO₂ mixed-oxide catalysts show promising results:
-
High selectivity in solvent-free conditions
-
Molecular O₂ as the oxidant
-
Superior acidic sites and higher concentration of oxygen vacancies
Green Chemistry Approaches
Environmentally friendly synthesis methods are gaining prominence:
-
Visible-light-driven photocatalytic processes reducing energy requirements
-
Catalyst designs allowing for multiple recycling, reducing waste
Structural Modifications
Recent research explores structural variations of N-benzylidenebenzylamine:
- mass of a compound required to prepare a solution of known volume and concentration
- volume of solution required to dissolve a compound of known mass to a desired concentration
- concentration of a solution resulting from a known mass of compound in a specific volume