L-beta-aspartyl-L-aspartic acid
CAS No.: 60079-22-3
Cat. No.: VC1968503
Molecular Formula: C8H12N2O7
Molecular Weight: 248.19 g/mol
* For research use only. Not for human or veterinary use.
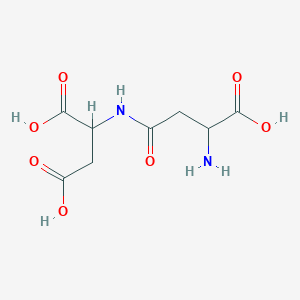
Specification
CAS No. | 60079-22-3 |
---|---|
Molecular Formula | C8H12N2O7 |
Molecular Weight | 248.19 g/mol |
IUPAC Name | 2-[(3-amino-3-carboxypropanoyl)amino]butanedioic acid |
Standard InChI | InChI=1S/C8H12N2O7/c9-3(7(14)15)1-5(11)10-4(8(16)17)2-6(12)13/h3-4H,1-2,9H2,(H,10,11)(H,12,13)(H,14,15)(H,16,17) |
Standard InChI Key | KXAWLANLJYMEGB-UHFFFAOYSA-N |
SMILES | C(C(C(=O)O)N)C(=O)NC(CC(=O)O)C(=O)O |
Canonical SMILES | C(C(C(=O)O)N)C(=O)NC(CC(=O)O)C(=O)O |
Introduction
Chemical Structure and Properties
L-beta-aspartyl-L-aspartic acid is a dipeptide formed by the linkage of two L-aspartic acid molecules through a beta-peptide bond. Unlike conventional peptides where amino acids connect via alpha-carboxyl groups, this compound features a connection between the beta-carboxyl group of the first aspartic acid and the alpha-amino group of the second aspartic acid.
Molecular Characteristics
The compound possesses distinctive chemical properties that differentiate it from standard dipeptides, as outlined in Table 1.
Table 1: Chemical Properties of L-beta-aspartyl-L-aspartic acid
Property | Value |
---|---|
Molecular Formula | C₈H₁₂N₂O₇ |
Average Molecular Weight | 248.19 g/mol |
Monoisotopic Molecular Weight | 248.064450748 |
IUPAC Name | 2-(3-amino-3-carboxypropanamido)butanedioic acid |
Traditional Name | 2-(3-amino-3-carboxypropanamido)butanedioic acid |
Physical State | Solid |
Solubility | Slightly soluble in water |
Acid-Base Property | Moderately acidic |
The molecular structure features multiple carboxylic acid groups and an amide linkage, contributing to its unique biochemical behavior in various physiological systems .
Nomenclature and Classification
This compound is known by several names and identifiers in scientific literature and chemical databases, reflecting its structural complexity and research significance.
Table 2: Nomenclature and Classification
Aspect | Description |
---|---|
Common Name | L-beta-aspartyl-L-aspartic acid |
Synonyms | beta-Aspartylaspartate, beta-Asp-Asp, b-Asp-Asp, 2-[(3-amino-3-carboxypropanoyl)amino]butanedioic acid |
CAS Registry Numbers | 1260642-13-4, 60079-22-3 |
Classification | Hybrid peptide |
Kingdom | Organic compounds |
Super Class | Organic acids and derivatives |
Class | Peptidomimetics |
Sub Class | Hybrid peptides |
Direct Parent | Hybrid peptides |
Family | N-acyl-alpha Amino Acids and Derivatives |
L-beta-aspartyl-L-aspartic acid belongs to the class of organic compounds known as hybrid peptides, which contain different types of amino acids (alpha, beta, gamma, delta) linked through peptide bonds .
Biochemical Significance
The compound plays crucial roles in several biochemical processes, particularly relating to protein modification, degradation, and metabolic pathways involving aspartic acid.
Formation and Occurrence
L-beta-aspartyl-L-aspartic acid primarily forms through two mechanisms:
-
Proteolytic breakdown of larger proteins containing aspartic acid residues
-
Spontaneous formation through non-enzymatic post-translational modifications of proteins
These processes are particularly prevalent in aging proteins or under conditions of cellular stress. The compound has been detected in various biological samples, including urine, and in food sources such as poultry (Anatidae, Gallus gallus), domestic pigs (Sus scrofa domestica), and cow milk .
Metabolic Pathway Integration
The metabolism of L-beta-aspartyl-L-aspartic acid intersects with several critical biochemical pathways. Its precursor, L-aspartic acid, participates in:
-
The urea cycle for nitrogen waste elimination
-
The purine-nucleotide cycle (PNC) for energy metabolism
-
The malate–aspartate shuttle (MAS), which is essential for maintaining NADH delivery to mitochondria and cellular redox balance
-
Gluconeogenesis pathways
-
Neurotransmission processes
The MAS system, in particular, represents a critical metabolic pathway involving aspartate. This shuttle consists of aspartate-glutamate carriers (AGC1/2) and 2-oxoglutarate carrier (OGC), along with enzymes including malate dehydrogenase (MDH) and aspartate aminotransferase (AST), found in both mitochondrial and cytosolic compartments .
Protein Modification and Aging
L-beta-aspartyl-L-aspartic acid formation is closely linked to protein aging and damage processes, representing significant biomarkers for protein deterioration.
Spontaneous Protein Modifications
Aspartic acid residues in proteins undergo various non-enzymatic modifications over time, including:
-
Deamidation - conversion of asparagine to aspartic acid
-
Isomerization - conversion between alpha and beta forms
-
Racemization - conversion between L and D stereoisomers
These modifications can result in the formation of unusual amino acid residues such as L-β-aspartyl, D-α-aspartyl, and D-β-aspartyl. Research has demonstrated these residues accumulate with age in long-lived proteins .
Kinetics of Modification
Studies on synthetic peptides have revealed important kinetic parameters of these modification processes. For example, the peptide L-Val-L-Tyr-L-Pro-L-Asn-Gly-L-Ala undergoes rapid deamidation with a half-life of only 1.4 days at 37°C and pH 7.4, forming an aspartyl succinimide intermediate. This intermediate further reacts through:
-
Hydrolysis (half-time: 2.3 hours)
-
Racemization (half-time: 19.5 hours)
The end products include a mixture of L- and D-normal aspartyl and beta-transpeptidation (isoaspartyl) hexapeptides. When asparagine is replaced by aspartic acid, the rate of succinimide formation decreases 34-fold .
Association with Age-Related Diseases
The accumulation of L-beta-aspartyl-L-aspartic acid and similar modified residues has been associated with various age-related conditions, including:
-
Cataracts (α-crystallin in the lens)
-
Neurodegenerative disorders (β-amyloid in the brain)
-
General protein dysfunction in aging tissues
These associations underline the potential clinical significance of understanding L-beta-aspartyl-L-aspartic acid formation and metabolism .
Enzymatic Interactions
Several enzymes interact with L-beta-aspartyl-L-aspartic acid, either in its formation, recognition, or repair processes.
Protein Repair Enzymes
Protein D-aspartyl (L-isoaspartyl) O-methyltransferase (PIMT) plays a critical role in protein repair by reverting unusual amino acid residues like L-β-aspartyl back to normal L-α-Asp conformations. PIMT catalyzes the transmethylation of S-adenosylmethionine to L-β-Asp and D-α-Asp residues in proteins and peptides .
Computational investigation of PIMT's substrate recognition mechanism has revealed that:
-
Hydrogen bonds of Ser60 and Val214 to the carboxyl group of Asp are crucial during substrate recognition
-
Specific hydrogen bonds form between the main chains of the substrates and those of Ala61 and Ile212 when PIMT recognizes L-β-Asp
-
Hydrophobic interactions between the (n-1) residue of substrates and Ile212/Val214 of PIMT significantly affect substrate binding
Beta-Aspartyl Peptidases
The NA23_RS08100 gene of Fervidobacterium islandicum AW-1 encodes a keratin-degrading β-aspartyl peptidase (FiBAP) that is highly expressed under starvation conditions. This enzyme exhibits maximal activity at 80°C and pH 7.0 in the presence of Zn²⁺. Structural analysis reveals it forms an octamer comprising a tetramer of dimers, with salt bridges contributing to its high thermostability .
Table 3: Enzymes Interacting with L-beta-aspartyl-L-aspartic acid
Enzyme | Function | Optimal Conditions | Significance |
---|---|---|---|
Protein D-aspartyl (L-isoaspartyl) O-methyltransferase (PIMT) | Reverts L-β-Asp to L-α-Asp | Physiological pH | Protein repair |
β-aspartyl peptidase (FiBAP) | Cleaves C-terminus of isoaspartyl residues | 80°C, pH 7.0, Zn²⁺ | Protein degradation under stress |
Aspartate aminotransferase | Amino group transfer from aspartate | Varies by isoform | Metabolism regulation |
L-asparaginase | Forms beta-aspartyl enzyme intermediate | Km ~60-160 μM | Protein modification |
These enzymes represent cellular mechanisms for managing damaged proteins containing non-standard aspartyl linkages .
Research Applications
The study of L-beta-aspartyl-L-aspartic acid has applications across multiple scientific disciplines.
Biomarker Development
L-beta-aspartyl-L-aspartic acid and similar modified aspartyl compounds show potential as biomarkers for:
-
Protein aging processes
-
Age-related diseases
-
Dietary intake of specific foods
-
Cellular stress conditions
Its detection in biological samples such as urine suggests applications in clinical diagnostics and metabolomics research .
Protein Repair Systems
Research on L-beta-aspartyl-L-aspartic acid has expanded understanding of cellular protein repair mechanisms. For example, expression of β-aspartyl peptidase can alleviate growth defects caused by heat shock-induced protein aggregates by degrading damaged proteins, suggesting therapeutic applications for conditions involving protein misfolding .
Pharmaceutical Development
Studies of aspartyl-containing compounds have contributed to pharmaceutical development. For instance, the substituted aspartate analogue L-beta-threo-benzyl-aspartate preferentially inhibits the neuronal excitatory amino acid transporter EAAT3, representing a potent and selective blocker for this transporter subtype. Such compounds demonstrate potential for:
Analytical Methods
Modern analytical techniques have enabled precise identification and quantification of L-beta-aspartyl-L-aspartic acid in complex biological matrices.
Detection Techniques
Various analytical methods have been employed for identifying L-beta-aspartyl-L-aspartic acid:
-
High-performance liquid chromatography (HPLC)
-
Mass spectrometry (MS), particularly LC-MS/MS
-
Nuclear magnetic resonance (NMR) spectroscopy
-
Fluorescence-based methods following derivatization
These techniques allow researchers to measure this compound in diverse biological samples with high sensitivity and specificity .
Structure Elucidation
Structural characterization of L-beta-aspartyl-L-aspartic acid has been accomplished through:
-
X-ray crystallography of protein-peptide complexes
-
NMR spectroscopy for solution-state confirmation
-
Computational modeling of molecular interactions
These approaches have clarified how this compound interacts with enzymes and other biological molecules .
Future Research Directions
Despite significant progress in understanding L-beta-aspartyl-L-aspartic acid, several research avenues remain unexplored.
Metabolic Integration
More comprehensive understanding is needed regarding:
-
The complete metabolic fate of L-beta-aspartyl-L-aspartic acid in various tissues
-
Regulatory mechanisms controlling its formation and degradation
-
Cross-talk between aspartyl modification pathways and other cellular processes
Such investigations would illuminate this compound's broader role in cellular metabolism .
- mass of a compound required to prepare a solution of known volume and concentration
- volume of solution required to dissolve a compound of known mass to a desired concentration
- concentration of a solution resulting from a known mass of compound in a specific volume