N-benzyl-2-methyl-4-nitroaniline
CAS No.: 201157-13-3
Cat. No.: VC1999923
Molecular Formula: C14H14N2O2
Molecular Weight: 242.27 g/mol
* For research use only. Not for human or veterinary use.
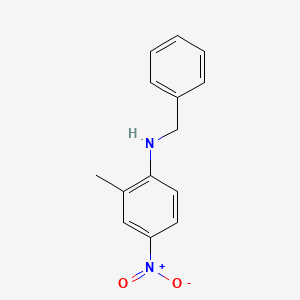
Specification
CAS No. | 201157-13-3 |
---|---|
Molecular Formula | C14H14N2O2 |
Molecular Weight | 242.27 g/mol |
IUPAC Name | N-benzyl-2-methyl-4-nitroaniline |
Standard InChI | InChI=1S/C14H14N2O2/c1-11-9-13(16(17)18)7-8-14(11)15-10-12-5-3-2-4-6-12/h2-9,15H,10H2,1H3 |
Standard InChI Key | WPJTZCLHFWRBBC-UHFFFAOYSA-N |
SMILES | CC1=C(C=CC(=C1)[N+](=O)[O-])NCC2=CC=CC=C2 |
Canonical SMILES | CC1=C(C=CC(=C1)[N+](=O)[O-])NCC2=CC=CC=C2 |
Introduction
Structural Properties
Basic Identification and Characteristics
N-benzyl-2-methyl-4-nitroaniline is an organic compound with the molecular formula C14H14N2O2 and a molecular weight of 242.27 g/mol . It is registered under CAS number 201157-13-3 and is characterized as a polar N-derivative of 2-methyl-4-nitroaniline (MNA) . In terms of physical appearance, BNA presents as a yellow powder at room temperature with a melting point of approximately 105°C .
Molecular Structure
The molecular structure of BNA consists of a 2-methyl-4-nitroaniline core with a benzyl group attached to the nitrogen atom. The compound's SMILES notation is represented as CC1=C(C=CC(=C1)N+[O-])NCC2=CC=CC=C2, while its InChI key is WPJTZCLHFWRBBC-UHFFFAOYSA-N . The structure features two benzene rings attached at an angle of approximately 80°, which significantly contributes to its unique optical and electronic properties .
Structural Identification Data
The following table summarizes the key structural identification parameters of BNA:
Property | Value |
---|---|
Molecular Formula | C14H14N2O2 |
Molecular Weight | 242.27 g/mol |
CAS Number | 201157-13-3 |
SMILES | CC1=C(C=CC(=C1)N+[O-])NCC2=CC=CC=C2 |
InChI | InChI=1S/C14H14N2O2/c1-11-9-13(16(17)18)7-8-14(11)15-10-12-5-3-2-4-6-12/h2-9,15H,10H2,1H3 |
InChI Key | WPJTZCLHFWRBBC-UHFFFAOYSA-N |
Melting Point | ~105°C |
Physical Appearance | Yellow powder at room temperature |
Synthesis Methods
Laboratory Synthesis Protocol
The synthesis of N-benzyl-2-methyl-4-nitroaniline involves a reaction between 2-methyl-4-nitroaniline (MNA) and benzyl bromide in the presence of specific reagents. The detailed procedure is as follows:
-
A mixture containing 20 g of 2-methyl-4-nitroaniline (MNA), 100 ml of hexamethyl phosphoric triamide (HMPA), 22 g of sodium bicarbonate, and 45 g of benzyl bromide is prepared in a round-bottom flask .
-
The entire solution is refluxed for 30 hours at 70°C under a nitrogen atmosphere .
-
After refluxing, 500 ml of double-distilled water is added to the solution .
-
The resulting mixture is extracted with diethyl ether and washed several times with saturated sodium chloride solution .
-
The organic layer is dried using anhydrous sodium sulfate powder, and the diethyl ether is evaporated to obtain BNA powder .
Purification Process
The synthesized BNA requires further purification to achieve high purity:
-
The crude BNA is purified by re-crystallizing it several times using high-performance liquid chromatography (HPLC)-grade methanol as a solvent .
-
The purification process is monitored using silica-gel thin layer chromatography (TLC) (n-hexane: ethyl acetate = 7:3) .
-
The final purified compound yields a single spot in TLC, confirming its purity .
Chemical Properties
Molecular Interactions
N-benzyl-2-methyl-4-nitroaniline demonstrates significant intermolecular interactions due to its unique structure. The aromatic structure of phenyl ligands in BNA enables efficient π-π strong interactions with other molecules, especially liquid crystals with cyanide (CN) groups . The phenyl-amine/methyl substitutes in BNA participate in strong intermolecular Coulomb interactions with the cyanide groups of liquid crystal molecules .
Optical Properties
BNA exhibits notable optical properties that make it valuable for various applications:
-
It possesses a relatively high nonlinear optics (NLO) coefficient (234 ± 31 pm/V) compared with other organic NLO materials .
-
BNA shows strong absorbance at approximately 345 nm, which enables it to act as a blue light intensity filter in liquid crystal devices .
-
The compound demonstrates significant potential for terahertz wave generation and nonlinear optical applications .
Theoretical Molecular Properties
Density Functional Theory (DFT) calculations provide insights into the molecular properties of BNA, as shown in the following table:
Property | BNA | 5CB (LC molecule) | 5CB + BNA |
---|---|---|---|
Dipole Moment (Debye) | 8.80 | 6.30 | 14.07 |
Polarizability (a.u.) | 207.99 | 238.29 | 446.15 |
Polarizability Anisotropy (a.u.) | 163.50 | 215.16 | 339.01 |
Energy Gap (eV) | 3.79 | 4.66 | 3.91 |
These calculations demonstrate that BNA increases the dipole moment, polarizability, and polarizability anisotropy of liquid crystal mixtures, which significantly impacts their dielectric anisotropy and electro-optical properties .
Applications in Liquid Crystal Technologies
Modification of Liquid Crystal Properties
One of the most significant applications of BNA is its use as a dopant in nematic liquid crystals (LCs). When dispersed in nematic LCs, BNA modifies several important properties:
-
Phase Transition Temperature: BNA doping decreases the nematic–isotropic phase transition temperature (TNI) of LC mixtures by approximately 12% .
-
Elastic Constants: The dispersion of BNA in LC mixtures reduces the splay elastic constant (K11) due to the decrease in order parameter (S) of the LC mixture .
-
Dielectric Anisotropy: BNA doping increases the dielectric anisotropy (Δε) of LC mixtures by approximately 6% .
-
Rotational Viscosity: BNA reduces the rotational viscosity (γ) of LC mixtures by up to 44%, which significantly impacts the response times of LC devices .
Preparation of BNA-Doped Liquid Crystal Mixtures
The preparation of BNA-doped liquid crystal mixtures typically follows these steps:
-
BNA powder is directly dissolved in liquid crystals without any solvents .
-
The mixture is ultrasonically stirred for 10-15 minutes at room temperature .
-
The prepared mixture is then introduced into liquid crystal cells through capillary action .
Electro-Optical Effects
Impact on Threshold Voltage
A significant electro-optical effect of BNA doping is the reduction in the threshold voltage (Vth) of liquid crystal cells. The threshold voltage is related to the splay elastic constant (K11) and dielectric anisotropy (Δε) through the following equation:
BNA doping decreases the threshold voltage by approximately 25% compared to pristine liquid crystal cells, primarily due to the reduced splay elastic constant and increased dielectric anisotropy of the LC mixture .
Effect on Response Times
One of the most remarkable effects of BNA doping is the significant improvement in the response times of liquid crystal cells, particularly the fall time (τoff). When operated in high voltage difference conditions, BNA-doped LC cells have a fall time that is five times faster than pure LC cells .
The fall time is related to the rotational viscosity (γ), cell thickness (d), and threshold voltage (Vth) through the following relationship:
The improved fall time results from three main factors:
-
Decreased threshold voltage (Vth) of the cell
-
Reduced rotational viscosity (γ) of the LC mixture
-
Additional restoring force provided by BNA's spontaneous polarization electric field (SPEF)
Parameter | Effect of 3 wt% BNA Doping | Mechanism |
---|---|---|
Threshold Voltage (Vth) | 25% decrease | Reduced splay elastic constant and increased dielectric anisotropy |
Dielectric Anisotropy (Δε) | 6% increase | Enhanced molecular interaction, dipole moment, and polarizability |
Rotational Viscosity (γ) | 44% decrease | Phenyl-methyl groups and decreased order parameter |
Fall Time (τoff) | 5× faster | Decreased threshold voltage, reduced viscosity, and additional restoring force from SPEF |
Rise Time (τon) | Decreased | Reduced rotational viscosity |
Comparative Studies
Comparison with Other Organic Dopants
A comparative study between BNA and Morpholinium 2-chloro-4-nitrobenzoate (M2C4N) reveals significant differences in their effects on liquid crystal properties. The following table presents a comparison of the electro-optical effects of these two organic dopants:
Electro-Optical Effect | BNA | M2C4N |
---|---|---|
Decrease in Threshold Voltage (Vth) | 25% | 20% |
Increase in Dielectric Anisotropy (Δε) | 6% | 16% |
Decrease in Rotational Viscosity (γ) | 44% | 25% |
Decrease in Fall Time (τoff) | 5× faster | 3× faster |
Coulomb Interaction with CN Group of LCs | Phenyl-amine/methyl | Phenyl-amine/hydroxyl/chloride |
Absorbance Peak of Dopant | 345 nm | 315 nm |
Absorbance Peak of Dopant-LC Mixture | 339 nm | 330 nm |
Analysis of Comparative Advantages
The comparative study indicates that each dopant offers specific advantages:
-
BNA Advantages:
-
Superior reduction in fall time (5× faster compared to 3× faster for M2C4N)
-
Greater decrease in rotational viscosity (44% vs. 25% for M2C4N)
-
Blue light filtering capability due to strong absorbance at ~345 nm
-
-
M2C4N Advantages:
-
Greater increase in dielectric anisotropy (16% vs. 6% for BNA)
-
Higher phase transition temperature in LC mixtures
-
The study concludes that M2C4N doping is more suitable for developing LC mixtures with high dielectric anisotropy, while BNA doping is more appropriate for fast-response and blue-light filtering LC devices .
Research Findings and Future Directions
Key Research Findings
Recent research has revealed several important findings regarding BNA:
-
Enhanced Electro-Optical Performance: The addition of BNA to nematic liquid crystals significantly enhances their electro-optical performance, particularly in terms of response time .
-
Molecular Mechanism: Density Functional Theory calculations have elucidated the molecular mechanism behind BNA's effects on LC mixtures, confirming that it strengthens the absorbance for blue light, enhances molecular interaction energy and dipole moment, decreases the molecular energy gap, and increases the permittivity of the LC mixture .
-
Blue Light Filtering: BNA doping exhibits strong absorbance at approximately 345 nm, which allows it to function as a blue light filter in LC devices, potentially offering additional benefits for display applications .
-
UV Protection Potential: The strong absorbance of BNA in the ultraviolet spectrum suggests potential applications in anti-UV technologies, such as smart windows and glasses .
Future Research Directions
Based on current findings, several promising directions for future research on BNA include:
-
Optimization of Concentration: Further studies to determine the optimal concentration of BNA for specific applications, balancing the improvements in response time against other properties.
-
Extended Applications: Exploration of BNA's potential in other optical and electronic applications beyond liquid crystal displays, particularly in nonlinear optics and terahertz wave generation.
-
Anti-UV Applications: Development of BNA-based materials for UV protection applications, leveraging its strong UV absorbance properties.
-
Novel Fabrication Techniques: Investigation of new methods for incorporating BNA into various materials and devices to maximize its beneficial effects.
- mass of a compound required to prepare a solution of known volume and concentration
- volume of solution required to dissolve a compound of known mass to a desired concentration
- concentration of a solution resulting from a known mass of compound in a specific volume