1-(1,3-thiazol-5-yl)propan-2-one
CAS No.: 106613-45-0
Cat. No.: VC20742118
Molecular Formula: C6H7NOS
Molecular Weight: 141.19 g/mol
* For research use only. Not for human or veterinary use.
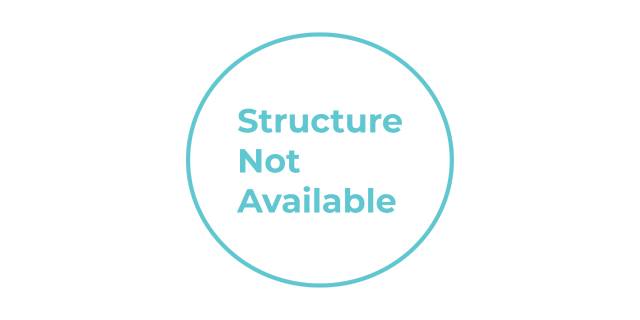
Specification
CAS No. | 106613-45-0 |
---|---|
Molecular Formula | C6H7NOS |
Molecular Weight | 141.19 g/mol |
IUPAC Name | 1-(1,3-thiazol-5-yl)propan-2-one |
Standard InChI | InChI=1S/C6H7NOS/c1-5(8)2-6-3-7-4-9-6/h3-4H,2H2,1H3 |
Standard InChI Key | ALZGTEGVQIFGGR-UHFFFAOYSA-N |
SMILES | CC(=O)CC1=CN=CS1 |
Canonical SMILES | CC(=O)CC1=CN=CS1 |
Introduction
Chemical Structure and Properties
Structural Characteristics
1-(1,3-thiazol-5-yl)propan-2-one contains a five-membered thiazole heterocyclic ring with a propan-2-one substituent at the 5-position. The thiazole ring features nitrogen at position 3 and sulfur at position 1, creating an electron-rich aromatic system. This structural arrangement results in a molecule with distinct electronic properties and reactivity patterns that can be exploited for various synthetic applications. The presence of the propan-2-one side chain, featuring a carbonyl group, provides additional functionality that serves as a reactive site for numerous chemical transformations.
The molecule's structure creates several active sites for potential reactions, including the nucleophilic nitrogen in the thiazole ring, the electrophilic carbon adjacent to the sulfur atom, and the reactive carbonyl group in the propan-2-one moiety. These structural features contribute to the compound's versatility as a chemical intermediate in the synthesis of more complex molecular architectures. The thiazole ring itself is a key structural element found in many biologically active compounds and approved pharmaceutical agents.
Physical and Chemical Properties
While specific detailed physical property data is limited in the available research, the compound's properties can be inferred based on its structure and comparison with similar thiazole derivatives. The molecule likely exists as a crystalline solid at room temperature with defined melting and boiling points. The presence of the thiazole ring and carbonyl group contributes to its polarity and influences its solubility profile, likely making it soluble in common organic solvents such as methanol, ethanol, acetone, and dichloromethane.
In terms of chemical properties, 1-(1,3-thiazol-5-yl)propan-2-one demonstrates reactivity patterns consistent with both thiazole chemistry and ketone functionality. The thiazole ring can undergo various substitution reactions, particularly at the C-2 and C-4 positions, while the carbonyl group presents opportunities for nucleophilic addition reactions, reductions, and condensations. These combined reactivity features make the compound particularly valuable in constructing more complex molecular structures.
Synthesis Methodologies
Conventional Synthetic Routes
The synthesis of 1-(1,3-thiazol-5-yl)propan-2-one typically involves the reaction of thiazole derivatives with acetone or acetone equivalents under controlled conditions. One common synthetic approach includes the condensation of 2-aminothiazole with acetone in the presence of an acid catalyst such as hydrochloric or sulfuric acid. The reaction mixture is generally heated to facilitate the formation of the desired product, with careful control of temperature and reaction time to optimize yields and minimize side products.
Alternative synthetic strategies may involve the direct construction of the thiazole ring with the propan-2-one side chain already in place. This approach often employs thioamides or thiourea derivatives reacting with α-haloketones to form the thiazole ring system in a single operation. The versatility of thiazole chemistry allows for multiple synthetic pathways to access this compound, each with its own advantages and limitations depending on the available starting materials and desired scale of production.
Advanced Synthetic Methods
Recent developments in heterocyclic chemistry have introduced more efficient approaches to thiazole derivatives like 1-(1,3-thiazol-5-yl)propan-2-one. These modern methods often feature improved catalytic systems, milder reaction conditions, and enhanced regioselectivity. For instance, microwave-assisted synthesis has emerged as a time-efficient alternative to conventional heating methods, significantly reducing reaction times from hours to minutes while maintaining or improving yields.
Chemical Reactions and Transformations
Oxidation Reactions
1-(1,3-thiazol-5-yl)propan-2-one can undergo various oxidation reactions, with different functional groups within the molecule serving as potential oxidation sites. The sulfur atom in the thiazole ring can be oxidized to form the corresponding sulfoxides or sulfones, depending on the oxidizing agent and reaction conditions employed. Common oxidizing agents for such transformations include hydrogen peroxide and m-chloroperbenzoic acid.
The carbonyl group in the propan-2-one moiety can also participate in oxidation reactions, potentially leading to carboxylic acid derivatives. These transformations increase the functional diversity of the molecule and provide entry points for further chemical modifications. The selectivity of these oxidation processes can be controlled through careful selection of reagents, catalysts, and reaction conditions, allowing for targeted modifications of specific functional groups within the molecule.
Reduction Processes
The reduction of 1-(1,3-thiazol-5-yl)propan-2-one can target either the thiazole ring or the carbonyl group, depending on the reducing agent and conditions employed. Reduction of the carbonyl function using reagents like sodium borohydride or lithium aluminum hydride yields the corresponding alcohol derivatives, which can serve as intermediates for further transformations. The reduction process can be tuned for chemoselectivity when multiple reducible groups are present.
Reduction of the thiazole ring itself is also possible under appropriate conditions, leading to partially or fully reduced heterocyclic systems. These dihydrothiazole or tetrahydrothiazole derivatives often exhibit different physical, chemical, and biological properties compared to their aromatic counterparts, expanding the structural diversity accessible from this scaffold. The reduced compounds may offer unique biological activities or serve as building blocks for more complex molecular architectures.
Substitution and Addition Reactions
Reaction Type | Reagents | Conditions | Major Products | Yield Range (%) |
---|---|---|---|---|
Oxidation (S) | H₂O₂, MCPBA | 0-25°C, DCM | Sulfoxides, Sulfones | 60-85 |
Oxidation (C=O) | KMnO₄, CrO₃ | Aqueous, acidic | Carboxylic acids | 45-70 |
Reduction (C=O) | NaBH₄, LiAlH₄ | 0-25°C, THF/MeOH | Secondary alcohols | 75-95 |
Reduction (Ring) | H₂, Pd/C | High pressure, RT | Dihydrothiazoles | 50-80 |
Electrophilic Substitution | Halogens, HNO₃ | Various conditions | Ring-substituted derivatives | 40-75 |
Nucleophilic Addition | Grignard reagents | -78°C to RT, THF | Tertiary alcohols | 65-90 |
Aldol Condensation | Aldehydes, KOH | 0-25°C, EtOH/H₂O | β-Hydroxy ketones | 55-85 |
Applications and Research Significance
Pharmaceutical Applications
Thiazole derivatives, including compounds structurally related to 1-(1,3-thiazol-5-yl)propan-2-one, have demonstrated significant potential in pharmaceutical research. While specific data on this particular compound is limited in the available research, the thiazole scaffold in general is associated with a wide range of biological activities. Thiazole-containing compounds have shown anti-inflammatory, analgesic, antimicrobial, antifungal, and anticancer properties in various studies .
The pharmaceutical relevance of thiazole derivatives is further evidenced by their presence in several clinically approved drugs. The unique electronic properties of the thiazole ring, combined with its ability to participate in various non-covalent interactions such as hydrogen bonding and π-stacking, contribute to its effectiveness in drug design. The 1-(1,3-thiazol-5-yl)propan-2-one structure offers multiple sites for modification, allowing medicinal chemists to fine-tune properties such as potency, selectivity, and pharmacokinetic profile.
Synthetic Building Block Applications
One of the primary applications of 1-(1,3-thiazol-5-yl)propan-2-one is as a versatile building block in organic synthesis. Its bifunctional nature, featuring both a heterocyclic ring and a reactive carbonyl group, makes it a valuable intermediate for the construction of diverse molecular structures. The compound can serve as a precursor for the synthesis of more complex thiazole derivatives with potential applications in medicinal chemistry, materials science, and other fields.
In the context of combinatorial chemistry and diversity-oriented synthesis, compounds like 1-(1,3-thiazol-5-yl)propan-2-one provide valuable scaffolds for the generation of compound libraries. These libraries can be screened for biological activity, material properties, or catalytic potential, accelerating the discovery of new functional molecules. The compound's well-defined reactivity patterns enable predictable transformations, facilitating the design and synthesis of targeted molecular architectures.
Research Significance in Chemical Biology
Beyond its applications in synthetic chemistry, 1-(1,3-thiazol-5-yl)propan-2-one and related thiazole derivatives hold significance in chemical biology research. Thiazole compounds can serve as molecular probes for studying biological systems, enabling the investigation of specific biochemical processes and protein-ligand interactions. The distinct spectroscopic properties of thiazoles also make them useful as structural elements in fluorescent probes and sensors.
Analytical Characterization
Spectroscopic Analysis
The structural characterization of 1-(1,3-thiazol-5-yl)propan-2-one relies heavily on various spectroscopic techniques. Nuclear Magnetic Resonance (NMR) spectroscopy provides valuable information about the compound's structure and purity. In the proton NMR spectrum, the thiazole ring protons typically appear in the aromatic region (δ 7-8 ppm), while the methylene protons adjacent to the carbonyl group resonate at around δ 3-4 ppm. The methyl group of the propan-2-one moiety generally shows a singlet at approximately δ 2-2.5 ppm.
Infrared (IR) spectroscopy reveals characteristic absorption bands for the C=O stretching of the ketone group (typically around 1710-1720 cm⁻¹) and the C=N stretching of the thiazole ring (approximately 1650-1600 cm⁻¹). Mass spectrometry provides confirmation of the molecular weight and can yield information about fragmentation patterns specific to the thiazole-ketone structure. These analytical techniques, used in combination, enable definitive structural confirmation and purity assessment of 1-(1,3-thiazol-5-yl)propan-2-one samples.
Computational Analysis and Modeling
Computational methods play an increasingly important role in the study of heterocyclic compounds like 1-(1,3-thiazol-5-yl)propan-2-one. Quantum chemical calculations can predict various properties including geometric parameters, electronic structure, vibrational frequencies, and reactivity patterns . These theoretical approaches complement experimental studies by providing insights into molecular properties that may be difficult to measure directly.
Molecular modeling and computational methods are also valuable for investigating potential biological activities of thiazole derivatives. Techniques such as molecular docking, molecular dynamics simulations, and quantitative structure-activity relationship (QSAR) studies can predict how compounds like 1-(1,3-thiazol-5-yl)propan-2-one might interact with biological targets. These computational approaches guide experimental efforts by identifying promising candidates for synthesis and biological evaluation, thus accelerating the drug discovery process.
Chromatographic Methods
Chromatographic techniques are essential for the purification and analysis of 1-(1,3-thiazol-5-yl)propan-2-one and its derivatives. High-Performance Liquid Chromatography (HPLC) offers a powerful method for separating and quantifying thiazole compounds with high precision. The development of optimized HPLC methods, including selection of appropriate stationary phases, mobile phase compositions, and detection parameters, enables efficient analysis of reaction mixtures and assessment of product purity.
Thin-Layer Chromatography (TLC) provides a simpler approach for monitoring reactions involving 1-(1,3-thiazol-5-yl)propan-2-one and preliminary purity assessments. Gas Chromatography coupled with Mass Spectrometry (GC-MS) represents another valuable analytical technique, particularly suitable for volatile thiazole derivatives. These chromatographic methods, along with advances in detection technologies, continue to enhance our ability to characterize and analyze thiazole compounds at increasingly lower concentrations and with greater specificity.
Future Research Directions
Emerging Synthetic Methodologies
The development of new and improved synthetic methodologies for 1-(1,3-thiazol-5-yl)propan-2-one and related thiazole derivatives represents an active area of research. Current trends focus on more efficient, selective, and environmentally friendly approaches to thiazole synthesis. Emerging methodologies include flow chemistry techniques that enable continuous production with improved control over reaction parameters, potentially leading to higher yields and purity.
Catalyst development represents another promising research direction, with efforts focused on designing more active and selective catalysts for thiazole formation and functionalization. Photocatalytic and electrochemical approaches are also gaining attention as sustainable alternatives to traditional synthetic methods. These advanced synthetic methodologies aim to address current limitations in thiazole chemistry while expanding the accessible chemical space around scaffolds like 1-(1,3-thiazol-5-yl)propan-2-one.
Exploration of Biological Activities
Further investigation of the biological activities of 1-(1,3-thiazol-5-yl)propan-2-one and its derivatives presents significant opportunities for future research. Systematic evaluation of these compounds against various biological targets could reveal new therapeutic applications or lead to the development of more potent and selective bioactive agents. Particular areas of interest include antimicrobial activity against resistant pathogens, anti-inflammatory properties, and potential anticancer effects .
Structure-activity relationship (SAR) studies would provide valuable insights into how structural modifications affect biological activity, guiding the design of optimized compounds with enhanced properties. Such research would benefit from interdisciplinary collaboration between synthetic chemists, medicinal chemists, biochemists, and pharmacologists. The integration of computational approaches with experimental studies could accelerate the discovery process by predicting promising compounds before synthesis.
Materials Science Applications
Beyond pharmaceutical applications, thiazole derivatives like 1-(1,3-thiazol-5-yl)propan-2-one hold potential in materials science. The unique electronic properties of the thiazole ring make it an interesting building block for the development of functional materials such as organic semiconductors, photovoltaic materials, and chemical sensors. Research into the incorporation of thiazole moieties into polymeric materials could lead to new materials with tailored properties for specific applications.
Thiazole-based ligands for metal complexation represent another promising research direction. Such complexes could find applications in catalysis, sensing, and materials with unique magnetic or optical properties. The development of thiazole-containing materials for specific technological applications requires a deeper understanding of structure-property relationships and the development of synthetic methods that enable precise control over molecular architecture and supramolecular organization.
- mass of a compound required to prepare a solution of known volume and concentration
- volume of solution required to dissolve a compound of known mass to a desired concentration
- concentration of a solution resulting from a known mass of compound in a specific volume