Erlotinib is an inhibitor of the epidermal growth factor receptor (EGFR) tyrosine kinase that is used in the treatment of non-small cell lung cancer, pancreatic cancer and several other types of cancer. It is typically marketed under the trade name Tarceva. Erlotinib binds to the epidermal growth factor receptor (EGFR) tyrosine kinase in a reversible fashion at the adenosine triphosphate (ATP) binding site of the receptor. Recent studies demonstrate that erlotinib is also a potent inhibitor of JAK2V617F, which is a mutant form of tyrosine kinase JAK2 found in most patients with polycythemia vera (PV) and a substantial proportion of patients with idiopathic myelofibrosis or essential thrombocythemia. This finding introduces the potential use of erlotinib in the treatment of JAK2V617F-positive PV and other myeloproliferative disorders.
Erlotinib is a Kinase Inhibitor. The mechanism of action of erlotinib is as a Protein Kinase Inhibitor.
Erlotinib is a tyrosine kinase receptor inhibitor that is used in the therapy of advanced or metastatic pancreatic or non-small cell lung cancer. Erlotinib therapy is associated with transient elevations in serum aminotransferase levels during therapy and rare instances of clinically apparent acute liver injury.
Erlotinib is a quinazoline derivative with antineoplastic properties. Competing with adenosine triphosphate, erlotinib reversibly binds to the intracellular catalytic domain of epidermal growth factor receptor (EGFR) tyrosine kinase, thereby reversibly inhibiting EGFR phosphorylation and blocking the signal transduction events and tumorigenic effects associated with EGFR activation.
A quinazoline derivative and ANTINEOPLASTIC AGENT that functions as a PROTEIN KINASE INHIBITOR for EGFR associated tyrosine kinase. It is used in the treatment of NON-SMALL CELL LUNG CANCER.
See also: Erlotinib Hydrochloride (has salt form).
Erlotinib
CAS No.: 183321-74-6
Cat. No.: VC20742481
Molecular Formula: C22H23N3O4
Molecular Weight: 393.4 g/mol
* For research use only. Not for human or veterinary use.
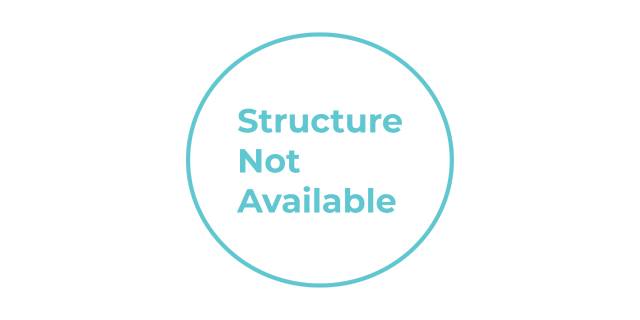
CAS No. | 183321-74-6 |
---|---|
Molecular Formula | C22H23N3O4 |
Molecular Weight | 393.4 g/mol |
IUPAC Name | N-(3-ethynylphenyl)-6,7-bis(2-methoxyethoxy)quinazolin-4-amine |
Standard InChI | InChI=1S/C22H23N3O4/c1-4-16-6-5-7-17(12-16)25-22-18-13-20(28-10-8-26-2)21(29-11-9-27-3)14-19(18)23-15-24-22/h1,5-7,12-15H,8-11H2,2-3H3,(H,23,24,25) |
Standard InChI Key | AAKJLRGGTJKAMG-UHFFFAOYSA-N |
SMILES | COCCOC1=C(C=C2C(=C1)C(=NC=N2)NC3=CC=CC(=C3)C#C)OCCOC |
Canonical SMILES | COCCOC1=C(C=C2C(=C1)C(=NC=N2)NC3=CC=CC(=C3)C#C)OCCOC |
Appearance | Powder |
Mechanism of Action
EGFR Signaling Pathway
The epidermal growth factor receptor (EGFR) signaling pathway plays a crucial role in cellular growth, proliferation, and survival. In normal cellular function, epidermal growth factor binds to EGFR, causing two EGFR molecules to form a homodimer . This dimerization enables the molecules to use ATP to trans-phosphorylate each other on tyrosine residues, generating phosphotyrosine residues that recruit phosphotyrosine-binding proteins . These proteins assemble complexes that transduce signal cascades to the nucleus or activate other cellular biochemical processes. In many cancers, particularly NSCLC, EGFR is overexpressed or contains activating mutations, leading to constitutive activation of these pathways and uncontrolled cellular proliferation.
Inhibitory Mechanism
Erlotinib specifically targets the EGFR tyrosine kinase by binding in a reversible fashion to the adenosine triphosphate (ATP) binding site of the receptor . When erlotinib binds to EGFR, the formation of phosphotyrosine residues becomes impossible, preventing the initiation of signal cascades necessary for cellular proliferation and survival . By blocking this critical step in EGFR signaling, erlotinib effectively inhibits the growth and proliferation of cancer cells that depend on this pathway, particularly those with EGFR mutations or overexpression. This targeted mechanism allows erlotinib to selectively affect cancer cells while sparing many normal cells, contributing to its therapeutic window.
Secondary Targets
Pharmacological Properties
Absorption and Bioavailability
Erlotinib is approximately 60% absorbed after oral administration, with its bioavailability substantially increased by food to almost 100% . Peak plasma levels occur approximately 4 hours after dosing, allowing for once-daily dosing schedules. The solubility of erlotinib is pH-dependent, decreasing as pH increases, which has implications for drug administration and potential interactions with medications that affect gastric pH . Additionally, smoking has been shown to decrease the exposure to erlotinib, which is an important consideration for patient populations with a history of smoking and may necessitate dose adjustments in current smokers to maintain therapeutic efficacy.
Distribution and Protein Binding
Erlotinib has an apparent volume of distribution of 232 L, indicating extensive tissue distribution beyond the vascular compartment . The drug is highly protein-bound, with approximately 93-95% binding to plasma proteins, primarily albumin and alpha-1 acid glycoprotein (AAG) . This high degree of protein binding affects the drug's distribution, metabolism, and elimination, and may influence its pharmacodynamic properties and potential for drug interactions with other highly protein-bound medications. The extensive tissue distribution may contribute to both the efficacy and toxicity profile of erlotinib in various organ systems.
Metabolism and Elimination
Erlotinib is primarily metabolized in the liver, with CYP3A4 serving as the main metabolizing enzyme and CYP1A2 playing a secondary role . The drug has a median elimination half-life of 36.2 hours, which supports its once-daily dosing regimen . More than 98% of erlotinib is excreted as metabolites, with over 90% eliminated via feces and approximately 9% via urine . This predominantly hepatic metabolism and biliary excretion pattern has important clinical implications for patients with liver dysfunction and potential drug-drug interactions with medications that induce or inhibit CYP enzymes, particularly CYP3A4.
Drug Interactions
Clinical Applications
Pancreatic Cancer
Erlotinib is also indicated for use in combination with gemcitabine as first-line treatment for patients diagnosed with locally advanced, unresectable, or metastatic pancreatic cancer . In this setting, the addition of erlotinib to gemcitabine has shown modest but statistically significant improvements in survival compared to gemcitabine alone. This application leverages the expression of EGFR in pancreatic cancer cells, although the response rates are generally lower than those observed in EGFR-mutated NSCLC. The combination therapy represents an important treatment option in a disease with historically limited therapeutic options and poor prognosis.
Clinical Trials and Efficacy Data
First-Line Therapy Studies
Studies of erlotinib as first-line therapy in unselected NSCLC populations have shown modest results. A phase II study by Giaccone et al. evaluated single-agent erlotinib in first-line therapy for patients with stage IIIB/IV NSCLC, reporting an objective response rate (ORR) of 22.7% and a disease control rate of 52.8% . Another phase II study by Lee et al. in patients ineligible for chemotherapy showed an ORR of 21% and a median OS of 3.2 months . These results suggest that patient selection is crucial for maximizing the benefit of first-line erlotinib therapy, with EGFR mutation status being the most important predictive biomarker for response.
Comparative Clinical Trial Data
Clinical trials have shown varying response rates and survival outcomes with erlotinib, as summarized in the following table from published literature:
Trial | Study phase | Treatment | Number of patients | ORR (%) | Median PFS/ TTP (months) | Median OS (months) |
---|---|---|---|---|---|---|
BR.21 [Shepherd et al. 2005] | Phase III | Erlotinib | N = 488 | 8.9 | 2.2 | 6.7 |
Placebo | N = 243 | <1 | 1.8 | 4.7 | ||
p < 0.001 | p < 0.001 | p < 0.001 | ||||
ISEL [Thatcher et al. 2005] | Phase III | Gefitinib | N = 1129 | 8 | 3.0 | 5.6 |
Placebo | N = 563 | 1.3 | 2.6 | 5.1 | ||
p < 0.0001 | p = 0.0006 | p = 0.089 |
Additionally, first-line studies with erlotinib and the related EGFR inhibitor gefitinib showed the following results:
Study | Study phase | Treatment | Number of patients | ORR (%) | Median PFS/TTP | Median OS |
---|---|---|---|---|---|---|
Giaccone et al.[2006] | Phase II | Erlotinib | 53 | 22.7 | 84 days | 391 days |
Lee et al.[2011] | Phase II | Erlotinib | 24 (ineligible for chemotherapy) | 21 | 1.5 months | 3.2 months |
Niho et al.[2006] | Phase II | Gefitinib | 40 | 30 | NA | 13.9 months |
Yang et al.[2008] | Phase II | Gefitinib | 106 | 50.9 | 5.5 months | 22.4 months |
These data demonstrate the variability in response to EGFR inhibitors across different patient populations and highlight the importance of proper patient selection .
Molecular Mechanisms of Cell Death
Apoptotic Pathways
Erlotinib induces cell death primarily through apoptotic pathways in cancer cells. In standard 2D monolayer NSCLC cultures, EGFR inhibition by erlotinib has been shown to induce Bcl-2 interacting mediator of cell death (BIM)- or reactive-oxygen species (ROS)-dependent apoptotic cell death . The interruption of EGFR signaling leads to downstream effects on various cellular pathways that ultimately culminate in programmed cell death. The specific molecular mechanisms involved in erlotinib-induced apoptosis continue to be an active area of research, with implications for understanding and potentially overcoming resistance mechanisms that may develop during treatment.
Autophagic Cell Death Mechanisms
Autophagy, a cell degradation pathway that can have either tumor suppressor or oncogenic roles in various cancer cells, has been reported to play an important role in EGFR inhibitor-induced cell death . There are conflicting reports about the role of autophagy in response to EGFR inhibition. Some studies suggest that autophagy initiated by EGFR inhibitors can up-regulate TRAIL (tumor necrosis factor-related apoptosis-inducing ligand) and induce apoptotic cell death, while others indicate that autophagy may suppress EGFR inhibitor-induced cell death in NSCLC . This complex interplay between autophagy and apoptosis represents an area of ongoing research with potential implications for developing strategies to enhance erlotinib efficacy.
Differences in 2D versus 3D Cell Models
Research has shown significant differences in the molecular mechanisms of erlotinib-induced cell death between 2D and 3D cell culture models. In 3D cultures, phosphorylation of EGFR is dramatically up-regulated compared to 2D cultures in certain NSCLC cell lines . Moreover, the rate of cell death in response to erlotinib is increased in 3D culture compared to 2D culture . These differences highlight the importance of considering the cellular microenvironment when studying drug mechanisms and may have implications for translating laboratory findings to clinical applications. The enhanced efficacy observed in 3D models suggests that traditional 2D cell culture systems may underestimate the potential clinical benefit of erlotinib and similar agents.
Mass Molarity Calculator
- mass of a compound required to prepare a solution of known volume and concentration
- volume of solution required to dissolve a compound of known mass to a desired concentration
- concentration of a solution resulting from a known mass of compound in a specific volume