Iodide has been investigated for the treatment of Goiter, Nodular.
I(-) is a metabolite found in or produced by Escherichia coli (strain K12, MG1655).
Iodide is a salt or ester of mineral iodine. Iodide salts are mild reducing agents and many react with oxygen to give iodine.
Inorganic binary compounds of iodine or the I- ion.
See also: Potassium Iodide (active moiety of); Sodium Iodide (active moiety of); Iodine I-120 (active moiety of).
Iodide ion
CAS No.: 100934-04-1
Cat. No.: VC20743273
Molecular Formula: I-
Molecular Weight: 126.9045 g/mol
* For research use only. Not for human or veterinary use.
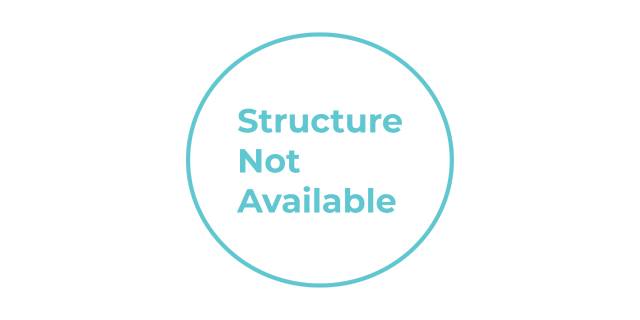
CAS No. | 100934-04-1 |
---|---|
Molecular Formula | I- |
Molecular Weight | 126.9045 g/mol |
IUPAC Name | iodide |
Standard InChI | InChI=1S/HI/h1H/p-1 |
Standard InChI Key | XMBWDFGMSWQBCA-UHFFFAOYSA-M |
SMILES | [I-] |
Canonical SMILES | [I-] |
Fundamental Properties of the Iodide Ion
Chemical Identity and Structure
The iodide ion (I-) represents the anionic form of elemental iodine. It is classified as a halide anion and monoatomic iodine species with a molecular weight of 126.9045 g/mol . As a negatively charged ion, iodide serves as the conjugate base of hydrogen iodide (HI) . Its chemical identity is well-established, with various synonyms including "iodide anion" and "trihydridoiodine" in scientific literature . The iodide ion's relatively large size compared to other halides influences its chemical behavior and reactivity patterns in both natural and synthetic environments.
Charge Characteristics and Electronic Configuration
The iodide ion carries a negative charge resulting from the acquisition of an additional electron compared to its neutral state . In its neutral form, the iodine atom possesses seven valence electrons. Through the gain of one electron, iodide achieves a stable octet configuration resembling that of noble gases . This electronic structure, with a complete outer shell of eight electrons, contributes to the ion's stability and its characteristic reactivity patterns in chemical reactions. The negative charge of the iodide ion is a fundamental aspect that determines its role in chemical processes, including its ability to form ionic compounds with various cations.
Physical and Chemical Properties
Iodide's physical and chemical properties derive largely from its ionic nature and electronic configuration. As one of the largest halide anions, iodide demonstrates distinctive chemical behavior compared to smaller halides like chloride and bromide. The iodide ion participates in various redox reactions, acting as a reducing agent in appropriate conditions. In aqueous solutions, iodide can be oxidized to elemental iodine or other iodine species depending on the oxidizing agent and reaction conditions. Its reactivity is influenced by factors such as temperature, pH, and the presence of other chemical species in solution.
Occurrence and Distribution
Natural Occurrence
Iodide occurs naturally in various environmental compartments, including seawater, groundwater, and certain mineral deposits. It exists as a component of several minerals, though generally in low concentrations compared to other halides. In biological systems, iodide serves as a human metabolite and has been identified as a metabolite in microorganisms such as Escherichia coli (strain K12, MG1655) . Its distribution in natural systems is influenced by geological processes, biological activity, and various chemical transformations.
Global Sea-Surface Iodide Distributions
Comprehensive studies have documented global sea-surface iodide concentrations across various oceanic regions. A significant dataset spanning from 1967 to 2018 includes 1342 data points covering latitudes from 70°S to 68°N and representing all major ocean basins . This compilation incorporates measurements from published manuscripts, directly supplied data from researchers, and repository records . The observed concentrations vary substantially, ranging from less than 10 to more than 200 nM across different marine environments . These variations reflect the complex biogeochemical cycling of iodine in the ocean, influenced by biological activity, physical oceanographic processes, and chemical transformations.
This extensive dataset supports advanced modeling of sea-surface iodide concentrations, facilitating research on processes such as ozone deposition to the sea surface and associated trace gas emissions to the atmosphere . Additionally, the data contributes to paleoceanographic research using iodine speciation as a tracer of past ocean oxygenation and helps predict the environmental impacts of anthropogenically released iodine radionuclides .
Biological Presence and Metabolic Role
Iodide plays critical roles in biological systems, particularly in human metabolism. It functions as an essential component of thyroid hormones, which regulate numerous physiological processes including metabolic rate, growth, and development. The thyroid gland actively concentrates iodide from the bloodstream to synthesize these hormones. Beyond humans, iodide serves metabolic functions in various organisms across different taxonomic groups, including certain bacteria where it has been identified as a metabolite . The ability of organisms to process and utilize iodide varies significantly, reflecting diverse evolutionary adaptations to this element.
Analytical Methods for Iodide Detection
Electrochemical Methods
Research indicates that approximately 75% of all data points collected using various analytical methods have a precision of 10% or better, while about 51% achieve a precision of 5% or better . These uncertainty levels are considered acceptable given the wide range of iodide concentrations observed globally, which span more than an order of magnitude from less than 10 to over 200 nM .
Ion Chromatography
Ion chromatography (IC) has emerged as a particularly effective method for iodide analysis, especially in pharmaceutical and quality control applications. This technique efficiently separates monovalent and divalent anions, including iodide, even in complex sample matrices . Research has demonstrated that a Metrosep A Supp 17 100/4.0 mm column with L91 packing provides optimal separation of iodide ions .
Two detection approaches have been evaluated for iodide assay: UV detection and conductivity detection after suppression . The latter method has proven more precise and accurate, with the additional advantage of simultaneously detecting other impurities such as chloride and sulfate . A fully validated ion chromatography method using suppressed conductivity detection has been developed for potassium iodide assay, meeting all validation criteria for specificity, linearity, system suitability, solution stability, accuracy, and precision .
The method demonstrates excellent linearity over a concentration range from 3.0 mg/L to 22.5 mg/L of iodide (20% to 150% of the expected concentration), with a correlation coefficient of 0.9999 . This analytical approach represents a significant improvement over traditional manual titration, which historically shows reduced precision and accuracy .
Applications and Significance
Medical Applications
Iodide compounds, particularly potassium iodide (KI), serve important therapeutic and preventive functions in medicine. Potassium iodide is used to treat hyperthyroidism (overactive thyroid) and to protect the thyroid gland from the effects of radiation exposure . Its effectiveness as a specific blocker of thyroid radioiodine uptake is well-established, reducing the risk of thyroid cancer in individuals or populations exposed to radioiodines through inhalation or ingestion . This protective function has significant public health implications, particularly in radiation emergency scenarios.
Additionally, iodide has been investigated for the treatment of goiter and nodular goiter . The therapeutic applications of iodide compounds highlight the importance of accurate and reliable methods for iodide assay in pharmaceutical preparations, which has driven developments in analytical techniques such as ion chromatography .
Environmental Significance
Iodide plays multiple roles in environmental processes, particularly in atmospheric chemistry and climate science. The dataset of global sea-surface iodide observations supports modeling of ozone deposition to the sea surface and associated trace gas emissions to the atmosphere . These processes have implications for atmospheric chemistry and climate dynamics.
Furthermore, iodide speciation serves as a valuable paleo-oceanographic tracer of past ocean oxygenation, contributing to our understanding of Earth's historical climate and ocean conditions . The environmental behavior of iodide is also relevant for predicting the impacts of iodine radionuclides released through anthropogenic activities . This application has significance for environmental risk assessment and management related to nuclear facilities and activities.
Recent Advances in Iodide Research
Iodide Ion Conductivity and Migration
Recent research has demonstrated significant iodide ion conduction in specialized solid materials, challenging previous assumptions about iodide's conductivity limitations. Studies on La₀.₇₀Sr₀.₂₅Zn₀.₀₅OI₀.₇₀ solid have quantified iodide ion migration, despite the traditional view that iodide is an inferior conductor due to its large ionic size compared to other conductive ion species .
Using modified Tubandt electrolysis, researchers observed weight increases at the anodic pellet and corresponding weight decreases at the cathodic pellet, consistent with theoretical values calculated for pure iodide ion migration . Further confirmation came from post-electrolysis analysis showing increased iodine concentration at the anode and decreased concentration at the cathode . This work represents the first study to definitively elucidate iodide ion conduction in solid materials.
The crystal structure of the conductive material features a layered arrangement along the c-axis, consisting of a rigid fluorite-type LaO layer and an iodide layer . In this structure, lanthanum ions are coordinated to four oxygen and four iodide ions, with the iodide ions arranged in a distinctive layer where they can migrate in the ab plane . The thermal and chemical stability of this lanthanum oxoiodide structure exceeds that of simple lanthanum iodide (LaI₃), making it particularly valuable for applied research .
Analytical Method Validation
Significant advances have been made in validating analytical methods for iodide determination. A fully validated ion chromatography method using suppressed conductivity detection has demonstrated excellent performance characteristics . The method validation included comprehensive assessment of specificity, linearity, system suitability, solution stability, accuracy, and precision . This approach provides a single chromatographic method for both assay and identification of iodide in potassium iodide, simplifying quality control workflows .
Parameter | Requirement | Result | Status |
---|---|---|---|
Column | N/A | Metrosep A Supp 17 100/4.0 (L91) | Compliant |
Flow Rate | N/A | 1.0 mL/min | Compliant |
Detection | N/A | Suppressed Conductivity | Compliant |
Tailing | NMT 2.0 for standard | 1.75 | Compliant |
Resolution | NLT 2.0 for resolution standard | 6.5 between phosphate and iodide | Compliant |
System Suitability | RSD NMT 1.0% for six replicate injections | 0.41% | Compliant |
Solution Stability | Change in peak NMT 1.0% from initial | Maximum 0.58 for standard and 0.77 for sample | Compliant |
Linearity | Correlation coefficient NLT 0.999 | 0.99999 | Compliant |
This validated method represents a significant improvement over traditional manual titration techniques, providing enhanced precision and accuracy for iodide determination in various matrices .
Mass Molarity Calculator
- mass of a compound required to prepare a solution of known volume and concentration
- volume of solution required to dissolve a compound of known mass to a desired concentration
- concentration of a solution resulting from a known mass of compound in a specific volume