Ribostamycin sulfate
CAS No.: 53797-35-6
Cat. No.: VC20753098
Molecular Formula: C17H36N4O14S
Molecular Weight: 552.6 g/mol
* For research use only. Not for human or veterinary use.
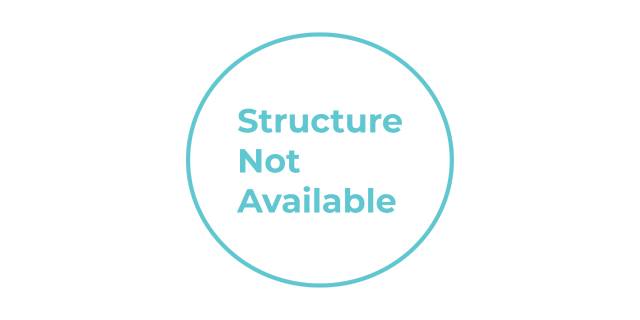
CAS No. | 53797-35-6 |
---|---|
Molecular Formula | C17H36N4O14S |
Molecular Weight | 552.6 g/mol |
IUPAC Name | (2R,3S,4R,5R,6R)-5-amino-2-(aminomethyl)-6-[(1R,2R,3S,4R,6S)-4,6-diamino-2-[(3R,4S,5R)-3,4-dihydroxy-5-(hydroxymethyl)oxolan-2-yl]oxy-3-hydroxycyclohexyl]oxyoxane-3,4-diol;sulfuric acid |
Standard InChI | InChI=1S/C17H34N4O10.H2O4S/c18-2-6-10(24)12(26)8(21)16(28-6)30-14-5(20)1-4(19)9(23)15(14)31-17-13(27)11(25)7(3-22)29-17;1-5(2,3)4/h4-17,22-27H,1-3,18-21H2;(H2,1,2,3,4)/t4-,5+,6-,7-,8-,9+,10-,11-,12-,13-,14-,15-,16-,17?;/m1./s1 |
Standard InChI Key | RTCDDYYZMGGHOE-CZUQXGEQSA-N |
Isomeric SMILES | C1[C@H]([C@@H]([C@H]([C@@H]([C@H]1N)O[C@@H]2[C@@H]([C@H]([C@@H]([C@H](O2)CN)O)O)N)OC3[C@@H]([C@@H]([C@H](O3)CO)O)O)O)N.OS(=O)(=O)O |
SMILES | C1C(C(C(C(C1N)OC2C(C(C(C(O2)CN)O)O)N)OC3C(C(C(O3)CO)O)O)O)N.[O-]S(=O)(=O)[O-] |
Canonical SMILES | C1C(C(C(C(C1N)OC2C(C(C(C(O2)CN)O)O)N)OC3C(C(C(O3)CO)O)O)O)N.OS(=O)(=O)O |
Chemical Properties and Structure
Molecular Composition
Ribostamycin sulfate is represented by the chemical formula C₁₇H₃₄N₄O₁₀ · H₂SO₄ or alternatively as C₁₇H₃₆N₄O₁₄S, with a molecular weight of 552.55 g/mol . The base compound ribostamycin itself has a formula of C₁₇H₃₄N₄O₁₀ with a molar mass of 454.477 g/mol .
Table 2.1: Chemical Properties of Ribostamycin Sulfate
Structural Characteristics
Ribostamycin possesses a complex structure comprised of three distinct ring subunits: 2-deoxystreptamine (DOS) serving as the central aminocyclitol core, neosamine C, and ribose . This tri-cyclic arrangement is fundamental to the compound's biological activity and specificity. The 2-deoxystreptamine core is particularly noteworthy as it is the most abundant aglycon core found in aminoglycoside antibiotics .
Physical Properties
The physical characterization of ribostamycin sulfate indicates that it should be stored at temperatures between 2-8°C to maintain stability . As with many aminoglycoside antibiotics, it exhibits water solubility due to its numerous hydroxyl and amine functional groups. This property facilitates its formulation for pharmaceutical applications and influences its pharmacokinetic behavior in biological systems.
Source and Isolation
Microbial Source
Ribostamycin is produced by Streptomyces ribosifidicus (also referred to as S. ribosidificus in some literature), a filamentous soil bacterium belonging to the actinomycetes family . This group of bacteria is renowned for its ability to produce a wide range of biologically active secondary metabolites, including many clinically important antibiotics. The original strain was isolated from soil collected in Tsu City of Mie Prefecture in Japan .
Isolation Procedures
Mechanism of Action
Interaction with Bacterial Ribosomes
Like other aminoglycoside antibiotics, ribostamycin exerts its antibacterial effect by binding to the 30S ribosomal subunit in bacterial cells . This interaction occurs at specific sites on the 16S ribosomal RNA component of the 30S subunit, interfering with the normal function of the bacterial protein synthesis machinery.
Effect on Protein Synthesis
The binding of ribostamycin to the 30S ribosomal subunit leads to two primary effects: misreading of the mRNA sequence during translation and inhibition of translocation . These disruptions effectively halt protein synthesis in susceptible bacteria, ultimately leading to cell death or growth inhibition. This mechanism of action is characteristic of the aminoglycoside class of antibiotics and underlies their bactericidal activity.
Spectrum of Activity
Ribostamycin demonstrates a broad spectrum of antibacterial activity, effectively targeting both gram-positive and gram-negative bacterial species . This wide-ranging efficacy makes it a valuable component of the antimicrobial arsenal. Additionally, research has indicated potential activity against HIV, expanding its therapeutic potential beyond bacterial infections .
Beyond its primary antimicrobial role, ribostamycin has been identified as an inhibitor of protein disulfide isomerase (PDI) , suggesting potential applications in areas outside infectious disease treatment. This multifaceted activity profile highlights the potential versatility of this compound in various therapeutic contexts.
Biosynthesis
Biosynthetic Pathway
The biosynthesis of ribostamycin follows a complex pathway beginning with the simple sugar D-glucose. The process initiates with the phosphorylation of D-glucose at the 6-position to form glucose-6-phosphate . From this starting material, a series of enzymatic transformations ultimately leads to the formation of the complete ribostamycin molecule. This biosynthetic route represents an elegant example of nature's ability to construct complex molecular architectures from simple precursors.
Key Enzymatic Reactions
Several enzymes play crucial roles in the biosynthesis of ribostamycin:
-
RbmA: This enzyme contains a genetic sequence corresponding to NAD+ binding and catalyzes the formation of 2-deoxy-scyllo-inosose from glucose-6-phosphate .
-
RmbB: Catalyzes the transamination of 2-deoxy-scyllo-inosose to 2-deoxy-scyllo-inosamine, utilizing L-glutamine and pyridoxal phosphate (PLP) as cofactors .
-
RbmC: Responsible for the oxidation of the ring to form 2-deoxy-3-amino-scyllo-inosose .
-
RmbB (second action): Performs another transamination reaction to convert 2-deoxy-3-amino-scyllo-inosose to 2-deoxystreptamine (DOS) .
-
RmbD: A glycosyltransferase that catalyzes the addition of sugar moieties to the DOS core .
This enzymatic cascade represents a remarkable example of nature's biosynthetic capabilities, converting simple precursors into complex, biologically active molecules through a coordinated series of chemical transformations.
Chemical Synthesis
Bottom-up Synthetic Approaches
Significant progress has been made in the total chemical synthesis of ribostamycin. A notable approach reported in the literature describes a bottom-up synthesis starting from benzene, which proceeds through ten linear operations to reach the final product . This synthetic achievement is particularly significant given the structural complexity of aminoglycoside antibiotics and the historically challenging nature of their synthesis.
Key Synthetic Steps
The reported synthetic route to ribostamycin involves several key transformations:
-
A hydroamination step that can be conducted on a 100 mmol scale, providing access to decagram quantities of a key intermediate .
-
A one-pot tert-butylcarbonate (Boc) protection and epoxidation sequence yielding an allylic epoxide as a single constitutional and diastereoisomer .
-
Formation of a bicyclic epoxide intermediate through a sequence involving a bromohydrin intermediate .
-
Incorporation of the final oxygen atom to the core using lithiated acetophenone ketoxime as a nucleophile .
-
Glycosylation with benzylated ribose to deliver a pseudodisaccharide with exclusive β-selectivity .
-
A second, α-selective glycosylation using a thioglycoside donor to afford the fully decorated pseudotrisaccharide .
-
Global deprotection involving the removal of six benzyl groups and conversion of azides and a bridgehead diazene to the corresponding amines, requiring Pearlman's catalyst under pressurized hydrogenation conditions (130 psi) .
This synthetic route represents a significant advancement in the chemical synthesis of complex aminoglycoside antibiotics, potentially opening avenues for the preparation of novel derivatives with enhanced properties.
Therapeutic Applications
Antibacterial Applications
The primary therapeutic application of ribostamycin sulfate lies in its broad-spectrum antibacterial activity. It is effective against a wide range of gram-positive and gram-negative bacterial pathogens . In clinical settings, aminoglycoside antibiotics like ribostamycin are often used in antimicrobial susceptibility tests (ASTs) to determine their efficacy against specific bacterial species . These tests help reduce the risk of using antibiotics against resistant bacteria and guide clinicians in selecting appropriate antimicrobial therapy.
Research Applications
Beyond its clinical applications, ribostamycin has proven valuable in various research contexts:
-
Pharmacokinetic studies: The compound has been used as a model drug in studies investigating the absorption, distribution, metabolism, and excretion properties of aminoglycoside antibiotics .
-
Kidney toxicity investigations: Ribostamycin has served as a tool in research examining nephrotoxicity, a common concern with aminoglycoside antibiotics .
-
As an inhibitor of protein disulfide isomerase (PDI): This property suggests potential applications in research on protein folding and related cellular processes .
Antibiotic Resistance
Mechanisms of Resistance
Resistance to aminoglycoside antibiotics like ribostamycin is a growing concern in clinical practice . Bacterial resistance to these antibiotics typically develops through enzymatic modifications of the antibiotic structure. These modifications occur through three main mechanisms:
-
Phosphorylation: Addition of phosphate groups to specific hydroxyl groups on the antibiotic molecule .
-
Acetylation: Addition of acetyl groups to amino functionalities .
These structural modifications prevent the antibiotic from effectively binding to its target site on bacterial ribosomal RNAs, thereby neutralizing its antibacterial activity.
Clinical Implications
The emergence of resistance to aminoglycoside antibiotics has significant implications for clinical practice. As resistance spreads, the efficacy of these important antimicrobial agents diminishes, potentially limiting treatment options for serious bacterial infections. This situation is particularly concerning given ribostamycin's classification as a critically important antimicrobial by the World Health Organization .
Strategies to Combat Resistance
Several approaches may help address the challenge of aminoglycoside resistance:
-
Development of synthetic derivatives with structural modifications that evade recognition by resistance enzymes.
-
Combination therapy with other classes of antibiotics to enhance efficacy and reduce the likelihood of resistance development.
-
Implementation of antimicrobial stewardship programs to promote appropriate use of these valuable antibiotics.
-
Continued research into novel antimicrobial agents and alternative approaches to combating bacterial infections.
Mass Molarity Calculator
- mass of a compound required to prepare a solution of known volume and concentration
- volume of solution required to dissolve a compound of known mass to a desired concentration
- concentration of a solution resulting from a known mass of compound in a specific volume