Ruthenium(III) chloride
CAS No.: 10049-08-8
Cat. No.: VC20874480
Molecular Formula: Cl₃Ru
Molecular Weight: 207.4 g/mol
* For research use only. Not for human or veterinary use.
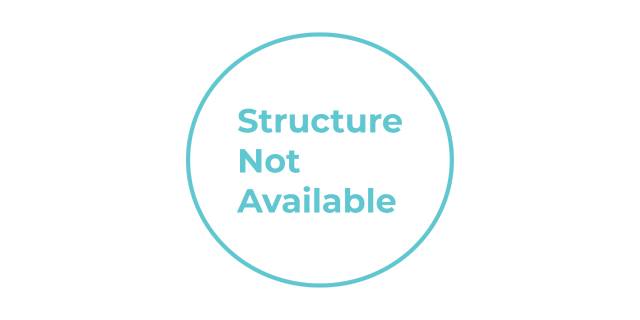
Specification
CAS No. | 10049-08-8 |
---|---|
Molecular Formula | Cl₃Ru |
Molecular Weight | 207.4 g/mol |
IUPAC Name | ruthenium(3+);trichloride |
Standard InChI | InChI=1S/3ClH.Ru/h3*1H;/q;;;+3/p-3 |
Standard InChI Key | YBCAZPLXEGKKFM-UHFFFAOYSA-K |
SMILES | [Cl-].[Cl-].[Cl-].[Ru+3] |
Canonical SMILES | [Cl-].[Cl-].[Cl-].[Ru+3] |
Introduction
Chemical Composition and Structure
Ruthenium(III) chloride exists in both anhydrous (RuCl₃) and hydrated (RuCl₃·xH₂O) forms. The hydrated form is more commonly used in laboratory applications and industrial processes. The compound has a molecular weight of 207.43 g/mol for the anhydrous form, while the hydrated form's weight varies depending on the degree of hydration . The molecular structure consists of a central ruthenium atom in the +3 oxidation state coordinated to three chloride ions, with varying numbers of water molecules in the hydrated form.
Physical and Chemical Properties
Ruthenium(III) chloride hydrate appears as a dark brown to black powder with specific physical and chemical characteristics that make it valuable for various applications. The compound is slightly soluble in water but dissolves well in hydrochloric acid. It decomposes in hot water and is insoluble in carbon disulfide and ethanol .
Table 1: Physical and Chemical Properties of Ruthenium(III) Chloride Hydrate
The compound is hygroscopic, meaning it readily absorbs moisture from the air, requiring careful storage to maintain its integrity . When dissolved in water, it forms an acidic solution with a pH below 2 .
Synthesis and Preparation Methods
Ruthenium(III) chloride can be synthesized through several methods, with the choice depending on the required purity and scale of production.
Industrial and Laboratory Synthesis
The compound can be prepared by direct combination of ruthenium metal and chlorine gas at high temperatures. Alternatively, it can be synthesized by adding hydrochloric acid to ruthenium hydroxide, followed by evaporation, concentration, and crystallization of the resulting mixture .
Purification Techniques
For high-purity applications, additional purification steps are employed. One common method involves dissolving the salt in water, filtering the solution, and concentrating it to crystallization in the absence of air to prevent oxidation. The solution can be evaporated in a stream of HCl gas while being heated just below its boiling point until a syrup is formed, followed by drying at 80-100°C under vacuum over H₂SO₄ .
For specialized applications requiring extremely high purity, heating at 700°C in the presence of Cl₂ produces the insoluble α-form of the compound . Commercial preparations are available in various purity grades, ranging from 99% (2N) to 99.999% (5N), catering to different application requirements .
Chemical Reactivity and Behavior
Ruthenium(III) chloride exhibits significant chemical reactivity that makes it valuable as a reagent and catalyst in various chemical transformations.
Reaction with Inorganic Compounds
The compound undergoes several characteristic reactions with inorganic substances:
-
It forms a precipitate when reacted with potassium iodide solution
-
Hydrogen sulfide causes formation of ruthenium trisulfide precipitate
-
It reacts with ammonia, potassium cyanide, and potassium nitrite to form corresponding ammonia, cyanide, and nitroso complexes
-
It can be reduced to blue divalent ruthenium ions by sodium amalgam or titanium trichloride
Behavior in Solution
In aqueous solutions, Ruthenium(III) chloride undergoes partial hydrolysis. Electrospray ionization mass spectrometry studies of similar ruthenium compounds have shown this hydrolysis process, confirming the dissociation of chloride ligands and/or solvent molecules . This behavior is typical for many ruthenium-based compounds, particularly the NAMI-type compounds, which undergo relatively quick dissociation of chloride ligands in solution.
Applications in Catalysis
Ruthenium(III) chloride serves as an essential catalyst for numerous chemical reactions, making it valuable in both academic research and industrial processes.
Organic Synthesis Applications
The compound is widely used as a catalyst for various organic transformations:
-
Oxidation reactions, especially selective oxidation of alcohols to corresponding aldehydes
-
Synthesis of 2-ethyl-3-methylquinolines from primary aromatic amines and triallylamine
-
Efficient catalyst for the synthesis of perimidine derivatives from ketones and naphthalene-1,8-diamine, using as little as 1 mol% of the catalyst
Industrial Catalytic Processes
In industrial settings, Ruthenium(III) chloride has proven effective in several catalytic applications:
-
When deposited on titania nanotubes, ruthenium hydrated oxide prepared from RuCl₃ can catalyze selective oxidation of alcohols by oxygen
-
It serves as a precursor for the preparation of various ruthenium-based catalysts, including the highly valuable Grubbs' catalysts used in olefin metathesis reactions
-
As a homogeneous catalyst for oxidation reactions of various organic substrates including valine, paracetamol, glycine, and other biologically relevant molecules
Research Applications
In research settings, the compound has been investigated for specialized catalytic applications:
-
As a catalyst for the oxidative cleavage of thiamine hydrochloride with N-bromosuccinimide in hydrochloric acid medium
-
In the kinetic study of oxidation of d-arabinose by N-bromophthalimide
-
For ruthenium-catalyzed oxidation of cyclamate with acidified bromamine-T
Biological and Medicinal Applications
Ruthenium(III) chloride and its complexes have garnered significant attention for their potential biological activities, particularly in anticancer and antimicrobial applications.
Anticancer Properties
Ruthenium(III) complexes derived from Ruthenium(III) chloride have demonstrated promising anticancer activities:
-
Six novel ruthenium(III) complexes with triazolopyrimidine derivatives have shown cytotoxic effects against human breast cancer MCF-7 cells and human cervical cancer HeLa cells
-
The ruthenium complex cis-(dichloro)tetrammineruthenium(III) chloride has exhibited cytotoxic effects on murine sarcoma 180 cells by inducing apoptosis and causing DNA damage
-
The most lipophilic complexes containing bulky ligands like dptp (5,7-diphenyl-1,2,4-triazolo[1,5-a]pyrimidine) have displayed particularly high cytotoxic activity against cancer cell lines
The mechanism of anticancer activity often involves the release of reactive oxygen species (ROS) intracellularly, which could indicate their role in triggering cell apoptosis . The interactions of these complexes with DNA, albumin, and apotransferrin have been extensively studied to understand their mode of action in biological systems.
Antimicrobial Activities
Ruthenium complexes have also shown promising antimicrobial properties:
-
Various Ru(II/III) complexes exhibit activity against pathogenic microorganisms through unique modes of action, including ROS generation, redox activation, ligand exchange, and depletion of substrates involved in vital cellular processes
-
The activity of these complexes against bacteria often depends on their lipophilicity and charge, affecting their ability to interact with specific targets like DNA, RNA, proteins, and bacterial membranes
-
The ruthenium ion provides these molecules with a positive charge, which aids in targeting the negatively charged cell wall structures of bacteria
Research has categorized ruthenium complexes as either stable, relatively inert compounds or prodrugs, with differing mechanisms of antimicrobial action:
-
Inert complexes maintain their ligand framework in biological media, with the ruthenium ion acting as a central scaffold for bioactive ligands
-
Prodrug complexes undergo chemical changes in biological environments, releasing active components
Structural Characterization of Ruthenium(III) Complexes
Understanding the structural features of Ruthenium(III) chloride and its complexes is crucial for developing applications and predicting their behavior in various environments.
X-ray Crystallography Data
X-ray diffraction studies have provided valuable insights into the structural characteristics of ruthenium(III) complexes. For example, novel ruthenium(III) complexes with histamine have been characterized by X-ray structure analysis, revealing protonated amino groups on the side chain of the ligand, which is uncommon for simple heterocyclic derivatives .
Table 2: Selected Crystal Structure Data for Ruthenium(III) Complexes
Parameter | Compound 1a | Compound 1b | NAMI Reference |
---|---|---|---|
Ru-Cl₁ (Å) | 2.355(2) | 2.359(2) | 2.3403(9) |
Ru-Cl₂ (Å) | 2.364(2) | 2.364(2) | 2.3227(8) |
Ru-Cl₃ (Å) | 2.356(2) | 2.343(2) | 2.3588(9) |
Ru-Cl₄ (Å) | 2.358(2) | 2.356(2) | 2.3447(8) |
Ru-S (Å) | 2.300(2) | 2.298(2) | 2.2956(6) |
Ru-N (Å) | 2.096(2) | 2.097(2) | 2.081(2) |
Note: Data from ruthenium complexes with histamine [RuCl₄(dmso-S)(histamineH)]·H₂O (1a) and [RuCl₄(dmso-S)(histamineH)] (1b) .
These structural studies reveal the distorted octahedral geometry commonly found in ruthenium(III) complexes, with bond lengths that can vary depending on the specific ligands and coordination environment.
Spectroscopic Characterization
Various spectroscopic techniques have been employed to characterize Ruthenium(III) chloride and its complexes:
-
Infrared (IR) spectroscopy
-
Electron Paramagnetic Resonance (EPR) spectroscopy
-
Mass spectrometry (particularly electrospray ionization mass spectrometry)
Mass spectrometry has proven particularly useful for understanding the behavior of ruthenium complexes in solution, detecting species formed through hydrolysis and ligand exchange processes. For example, in one study, peaks detected at m/z values of 264, 299, 325, and 361 were assigned to fragments like RuCl(histamine)(OH), RuCl₂(histamine)(OH), RuCl(dmso)(histamine), and RuCl₂(dmso)(histamine), respectively .
Purity Grade | Ruthenium Content | Form | Color |
---|---|---|---|
(2N) 99% | 35-40% | Powder | Dark brown to black |
(3N) 99.9% | 35-40% | Powder | Dark brown to black |
(4N) 99.99% | 35-40% | Powder | Dark brown to black |
(5N) 99.999% | 35-40% | Powder | Dark brown to black |
Data compiled from commercial suppliers .
Element | Maximum Limit |
---|---|
Calcium (Ca) | ≤300 ppm |
Copper (Cu) | ≤300 ppm |
Gold (Au) | ≤300 ppm |
Iridium (Ir) | ≤500 ppm |
Iron (Fe) | ≤300 ppm |
Osmium (Os) | ≤100 ppm |
Palladium (Pd) | ≤500 ppm |
Platinum (Pt) | ≤500 ppm |
Rhodium (Rh) | ≤500 ppm |
Silver (Ag) | ≤300 ppm |
Sodium (Na) | ≤300 ppm |
Values based on trace analysis with respect to ruthenium content .
- mass of a compound required to prepare a solution of known volume and concentration
- volume of solution required to dissolve a compound of known mass to a desired concentration
- concentration of a solution resulting from a known mass of compound in a specific volume