Beryllium oxide is an odorless white solid. Sinks in water. (USCG, 1999)
Beryllium oxide is a beryllium molecular entity consisting of beryllium (+2 oxidation state) and oxide in the ratio 1:1. In the solid state, BeO adopts the hexagonal wurtzite structure form while in the vapour phase, it is present as discrete diatomic covalent molecules. It has a role as a carcinogenic agent. It is a beryllium molecular entity and a metal oxide.
Beryllium oxide
CAS No.: 1304-56-9
Cat. No.: VC20938129
Molecular Formula: BeO
Molecular Weight: 25.012 g/mol
* For research use only. Not for human or veterinary use.
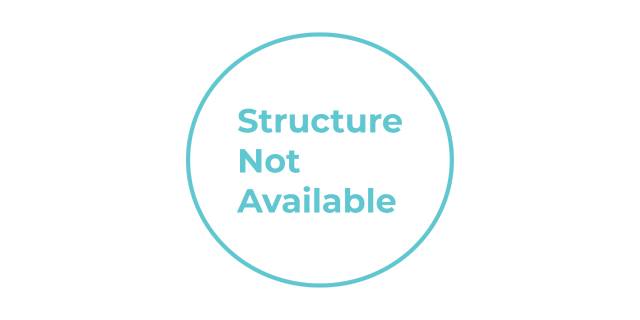
Specification
CAS No. | 1304-56-9 |
---|---|
Molecular Formula | BeO |
Molecular Weight | 25.012 g/mol |
IUPAC Name | oxoberyllium |
Standard InChI | InChI=1S/Be.O |
Standard InChI Key | LTPBRCUWZOMYOC-UHFFFAOYSA-N |
Impurities | Beryllium oxide-purities: 99-99.99% ... The purity of beryllia is critical to its thermal conductivity: as the purity drops below 99.5%, thermal conductivity drops off rapidly. Impurities (mg/kg): Al, 46; Fe, 32; Cr, 8; Mn, < 2; Ni, 9; B, 2; Ca, 31; Co, < 1; Cu, 3; Si, 1861; Mg, 922; Li, 2; Zn, < 20; Ti, 5; Na, 173; Ag, < 1; Mo, < 3; Pb, 2. Silicon and magnesium silicates are added to beryllia powder as sintering aids. |
SMILES | [Be]=O |
Canonical SMILES | [Be]=O |
Boiling Point | 3787 °C |
Colorform | White hexagonal crystals Light, amorphous powder White, amorphous powder or white hexagonal crystals |
Melting Point | 2578 °C 2530 °C |
Introduction
Historical Context
The history of beryllium oxide is closely tied to the discovery and utilization of beryllium compounds. The sweet taste of beryllium compounds led to early naming conventions, with beryllium oxide being called glucina or glucinium oxide . This characteristic sweet taste, while historically notable, also highlights the significant health concerns associated with beryllium compounds, as they are now known to be toxic.
Research interest in beryllium oxide intensified during the mid-20th century, particularly as its potential applications in nuclear technology and electronics became apparent. The scientific paper from the National Bureau of Standards demonstrates the detailed thermodynamic studies conducted on beryllium oxide by the 1960s, indicating its increasing importance in scientific and industrial applications .
Preparation and Chemical Properties
Synthesis Methods
Beryllium oxide can be prepared through several established chemical processes. The primary methods of synthesis include:
-
Calcination of beryllium carbonate, which decomposes to form beryllium oxide and carbon dioxide:
BeCO₃ → BeO + CO₂ -
Dehydration of beryllium hydroxide, yielding beryllium oxide and water:
Be(OH)₂ → BeO + H₂O -
Direct oxidation of metallic beryllium in air, producing beryllium oxide:
2 Be + O₂ → 2 BeO
It should be noted that when beryllium is ignited in air, the reaction may produce a mixture of beryllium oxide and beryllium nitride (Be₃N₂) rather than pure BeO . This necessitates additional purification steps for applications requiring high-purity beryllium oxide.
For industrial and research applications, the synthesis process must be carefully controlled to achieve the desired purity and physical characteristics. The research described in the scientific literature details the preparation of beryllium oxide samples with different densities (2.3 g/cm³ and 1.6 g/cm³) through pressing, firing, and sintering BeO powder at different temperatures (1,800°C and 1,100°C respectively) .
Chemical Behavior
-
A solution of hot concentrated sulfuric acid (H₂SO₄) combined with ammonium sulfate ((NH₄)₂SO₄)
The chemical reactivity of beryllium oxide is significantly influenced by its preparation conditions, particularly the temperature at which it is formed. This temperature-dependent behavior has important implications for its processing, applications, and safety considerations.
Crystal Structure
Beryllium oxide crystallizes in the hexagonal wurtzite structure, which features tetrahedral Be²⁺ and O²⁻ centers . This crystal structure is similar to that of lonsdaleite (hexagonal diamond) and hexagonal boron nitride (w-BN), with which beryllium oxide is isoelectronic .
The crystallographic arrangement of beryllium oxide differs significantly from the oxides of larger Group 2 metals (magnesium, calcium, strontium, and barium). While BeO adopts the hexagonal wurtzite structure, MgO, CaO, SrO, and BaO crystallize in the cubic rock salt motif with octahedral geometry around the dications and dianions .
At elevated temperatures, beryllium oxide undergoes a phase transformation from its standard hexagonal structure to a tetragonal form . Additionally, a phase change in BeO has reportedly been observed at approximately 2,220°K .
In the vapor phase, beryllium oxide exists as discrete diatomic molecules. These gas-phase molecules can be described using valence bond theory as adopting sp orbital hybridization on both atoms, featuring one σ bond (between one sp orbital on each atom) and one π bond (between aligned p orbitals on each atom oriented perpendicular to the molecular axis) .
Thermodynamic Properties
Enthalpy and Heat Capacity
Detailed thermodynamic studies of beryllium oxide have been conducted to characterize its enthalpy, heat capacity, entropy, and Gibbs free-energy function across various temperature ranges. Research at the National Bureau of Standards measured the enthalpy of high-purity sintered beryllium oxide relative to 273.15°K, up to temperatures of 1,173°K using a Bunsen ice calorimeter and the drop method .
The enthalpy of beryllium oxide can be represented by the following empirical equation (in cal mole⁻¹ at T°K) :
H°ₜ-H°₂₇₃.₁₅ = 11.1084T + 7.1245(10⁻⁴)T² + 8.40705(10⁵)T⁻¹ - 5.31245(10⁷)T⁻² - 5453.21
This equation enables the calculation of enthalpy values across a wide temperature range with high precision. The heat capacity (Cp) of beryllium oxide increases with temperature, as shown in the table below derived from the research data :
Table 3.1: Heat Capacity and Thermodynamic Properties of Beryllium Oxide
Temperature (°K) | Cp (cal deg⁻¹ mole⁻¹) | H°ₜ-H°₂₉₈.₁₅ (cal mole⁻¹) |
---|---|---|
400 | 10.310 | 1046.5 |
500 | 10.939 | 2107.3 |
600 | 11.324 | 4382.2 |
700 | 11.643 | 5531.3 |
800 | 11.891 | 6708.5 |
900 | 12.090 | 7907.8 |
1000 | 12.255 | 9125.4 |
1100 | 12.391 | 10358 |
1200 | 12.505 | 11603 |
The heat capacity data show a gradual increase with temperature, which is typical for many ceramic materials. This progressive rise in heat capacity reflects the increasing vibrational energy states that become accessible as temperature increases. |
Comparative Analysis with Similar Compounds
Comparative studies between beryllium oxide and other similar compounds, particularly magnesium oxide and aluminum oxide, reveal significant differences in their thermodynamic behavior and physical properties. The research conducted at the National Bureau of Standards included parallel measurements of magnesium oxide, allowing for direct comparison .
Beryllium oxide exhibits thermal conductivity ten times higher than alumina (Al₂O₃) and fifty percent higher than aluminum nitride (AlN) . This exceptional thermal conductivity distinguishes BeO from other ceramic materials and makes it particularly valuable for thermal management applications.
The density of beryllium oxide (3.01 g/cc) is significantly lower than that of alumina (4.00 g/cc) and somewhat lower than aluminum nitride (3.26 g/cc) . This lower density provides weight advantages in applications where mass is a consideration.
When comparing the dielectric properties, beryllium oxide exhibits a lower dielectric constant than both alumina and aluminum nitride . This characteristic makes it particularly suitable for high-frequency electronic applications where low dielectric loss is essential.
Applications and Usage
Electronics and Thermal Management
Perhaps the most significant commercial application of beryllium oxide is in electronic components and thermal management systems. BeO's extraordinary combination of high thermal conductivity and electrical insulation makes it uniquely suited for substrates and heat spreaders in high-power electronic devices .
Beryllium oxide is employed in thermal management systems for integrated electronics, where it efficiently conducts heat away from sensitive components while maintaining electrical isolation . This capability is crucial in high-performance computing, power electronics, and telecommunications equipment where heat dissipation is a critical design consideration.
The material's low dielectric constant and excellent dielectric loss characteristics further enhance its suitability for high-frequency applications, including radio frequency (RF) and microwave components . In these applications, BeO substrates help maintain signal integrity while managing the thermal challenges associated with high-frequency operation.
Nuclear Applications
Beryllium oxide has significant applications in nuclear technology, serving as a high-temperature, high-performance refractory component for nuclear reactors . Its combination of properties makes it particularly valuable in this demanding environment.
In nuclear applications, BeO can function as a moderator material, neutron reflector, or structural component. Its low atomic mass makes it effective at slowing down (moderating) neutrons without capturing them, which is beneficial for certain reactor designs. Additionally, its high thermal conductivity helps manage the intense heat generated in nuclear reactions.
The research and development partnerships mentioned by American Beryllia Inc. include universities engaged in advanced materials research, suggesting ongoing development of BeO applications in cutting-edge fields . These collaborations highlight the continued relevance and evolution of beryllium oxide applications in high-technology sectors.
- mass of a compound required to prepare a solution of known volume and concentration
- volume of solution required to dissolve a compound of known mass to a desired concentration
- concentration of a solution resulting from a known mass of compound in a specific volume