Dysprosium oxide
CAS No.: 1308-87-8
Cat. No.: VC20954067
Molecular Formula: Dy2O3
Molecular Weight: 372.9982
* For research use only. Not for human or veterinary use.
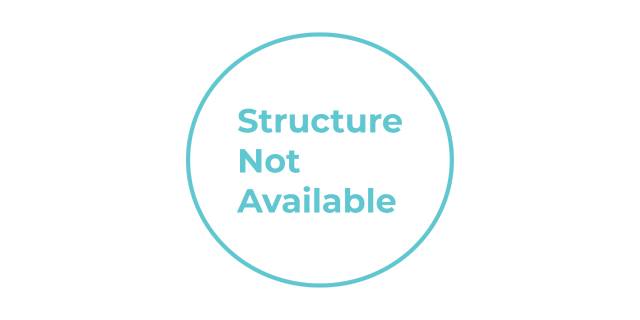
CAS No. | 1308-87-8 |
---|---|
Molecular Formula | Dy2O3 |
Molecular Weight | 372.9982 |
IUPAC Name | dysprosium(3+);oxygen(2-) |
Standard InChI | InChI=1S/2Dy.3O/q2*+3;3*-2 |
Standard InChI Key | GEZAXHSNIQTPMM-UHFFFAOYSA-N |
SMILES | [O-2].[O-2].[O-2].[Dy+3].[Dy+3] |
Canonical SMILES | [O-2].[O-2].[O-2].[Dy+3].[Dy+3] |
Physical and Chemical Properties
Physical Properties
Dysprosium oxide possesses several distinctive physical properties that contribute to its technological significance. Table 1 summarizes the key physical characteristics of this compound.
Chemical Properties
Dysprosium oxide demonstrates important chemical properties and participates in various reactions. When dysprosium metal is exposed to oxygen, it readily forms dysprosium oxide through the following reaction:
4 Dy + 3 O₂ → 2 Dy₂O₃
The compound exhibits basic oxide characteristics, reacting with acids to form corresponding dysprosium salts. For instance, dysprosium metal dissolves in dilute sulfuric acid to produce dysprosium(III) sulfate:
2 Dy + 3 H₂SO₄ → 2 Dy³⁺ + 3 SO₄²⁻ + 3 H₂
Dysprosium oxide itself reacts with acids in similar fashion. When exposed to moist air, dysprosium metal reacts slowly to form dysprosium hydroxide, which can further decompose at elevated temperatures to form dysprosium oxide:
2 Dy(OH)₃ → Dy₂O₃ + 3 H₂O
The compound is slightly hygroscopic, absorbing moisture from the air, which affects its handling and storage requirements .
Thermodynamic Properties
While comprehensive thermodynamic data specifically for dysprosium oxide is limited in the available literature, the thermodynamic properties of elemental dysprosium provide valuable context. Table 2 presents key thermodynamic values for dysprosium metal, which is relevant to understanding dysprosium oxide behavior.
Synthesis Methods
Conventional Synthesis
Traditional synthesis of dysprosium oxide typically involves the calcination of dysprosium-containing precursors such as dysprosium hydroxide, dysprosium carbonate, or dysprosium nitrate. The general process entails heating these precursors to high temperatures, triggering decomposition and the formation of dysprosium oxide.
The decomposition pathway generally follows these steps:
Dy(OH)₃ → DyO(OH) + H₂O
2 DyO(OH) → Dy₂O₃ + H₂O
This conventional approach remains widely used for industrial-scale production of dysprosium oxide for various applications.
Nanoparticle Synthesis
With advancing nanotechnology, several methods have been developed to synthesize dysprosium oxide nanoparticles, which offer enhanced properties due to their high surface area-to-volume ratio.
One sophisticated approach involves a hydrothermal method for creating Dy₂O₃@TiO₂ nanocomposites. This process begins with mixing dysprosium nitrate (Dy(NO₃)₃·6H₂O) with sodium hydroxide to form a white suspension. Titanium tetraisopropoxide and additional sodium hydroxide are then added while stirring constantly. The mixture is sealed in a Teflon-lined stainless-steel autoclave and heated at 180°C for 24 hours. After filtration, washing, and drying, the precipitate is calcined at 550°C for 4 hours to produce the final Dy₂O₃@TiO₂ nanocomposite .
Another innovative method employs organic polymers with nanosized pores to create dysprosium oxide nanoparticles. This process includes:
-
Preparing a dysprosium salt solution by dissolving the salt in an appropriate solvent
-
Immersing an organic polymer with nanosized pores (1-9 nm) in the solution
-
Heating the polymer with the immersed salt until the organic polymer is calcined
This approach allows for precise control of nanoparticle size, typically yielding particles in the tens of nanometers range, which are particularly valuable for specialized applications .
Applications of Dysprosium Oxide
Magnetic Applications
Dysprosium oxide exhibits remarkable paramagnetic properties that make it invaluable for various magnetic applications. It serves as a key raw material for producing dysprosium metal, which is essential in manufacturing high-performance magnets, particularly neodymium-iron-boron (NdFeB) permanent magnets .
The addition of approximately 2-3% dysprosium to NdFeB magnets significantly enhances their coercive force—the ability to resist demagnetization. This property is crucial for applications requiring stable magnetic performance under varying conditions, such as electric vehicles, wind turbines, and computer hard drives .
Additionally, dysprosium oxide is utilized in magneto-optical recording materials, which are employed in techniques to write, read, erase, and store data using laser and magnetic technologies .
Biomedical Applications
The biomedical potential of dysprosium oxide, particularly in nanoparticle form, has gained significant attention in recent research. The fluorescence and paramagnetic properties of dysprosium oxide nanoparticles (nDy₂O₃) make them promising candidates for:
Nuclear Applications
Dysprosium oxide has important applications in nuclear technology due to its exceptional neutron absorption properties. It is utilized in:
-
Measurement of neutron energy spectrum
-
Nuclear reaction control rods
-
Neutron absorbers
These applications leverage dysprosium's ability to capture neutrons efficiently, making it valuable for controlling nuclear reactions and for sophisticated neutron detection and measurement systems.
Recent Research Developments
Nanocomposites
Current research has focused intensively on developing dysprosium oxide-based nanocomposites with enhanced functional properties. A notable example is the synthesis of Dy₂O₃@TiO₂ nanocomposites using a single-step hydrothermal approach for advanced photocatalytic and electrocatalytic applications. These innovative nanocomposites combine the properties of both dysprosium oxide and titanium dioxide to achieve substantially improved performance in various catalytic processes .
The synthesis methodologies for these nanocomposites have been refined to ensure optimal integration of the components, resulting in materials with enhanced surface area, improved reactivity, and superior stability under demanding operational conditions.
Environmental and Toxicological Studies
With the increasing use of dysprosium oxide nanoparticles in various applications, understanding their environmental impact and potential toxicity has become a critical research focus. Comprehensive studies have evaluated the effects of dysprosium oxide nanoparticles on biological systems under different environmental conditions .
Research has employed advanced toxicity assessment techniques, including fluorescent dyes (Live/Dead) and respirometric assays, to measure cellular responses such as undisturbed cell membrane integrity and remaining respiration percentage. These studies have provided valuable insights into the mechanisms of toxicity and the factors that influence the biological effects of dysprosium oxide nanoparticles .
The findings suggest that environmental conditions, including salt and nutrient concentrations, significantly affect the toxicity profile of dysprosium oxide nanoparticles. This understanding is crucial for developing appropriate safety guidelines and mitigation strategies as the use of these materials continues to expand across various industries.
Mass Molarity Calculator
- mass of a compound required to prepare a solution of known volume and concentration
- volume of solution required to dissolve a compound of known mass to a desired concentration
- concentration of a solution resulting from a known mass of compound in a specific volume