Lutetium(III) iodide
CAS No.: 13813-45-1
Cat. No.: VC21002371
Molecular Formula: I3Lu
Molecular Weight: 555.6802 g/mol
* For research use only. Not for human or veterinary use.
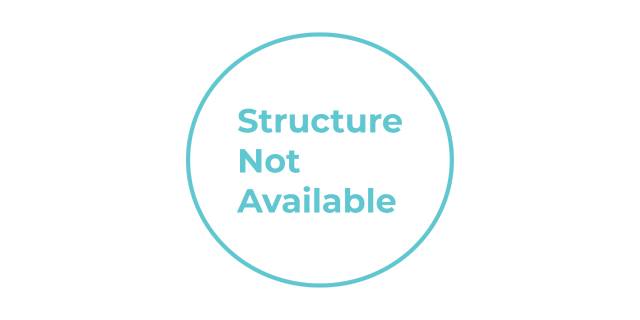
Specification
CAS No. | 13813-45-1 |
---|---|
Molecular Formula | I3Lu |
Molecular Weight | 555.6802 g/mol |
IUPAC Name | lutetium(3+);triiodide |
Standard InChI | InChI=1S/3HI.Lu/h3*1H;/q;;;+3/p-3 |
Standard InChI Key | NZOCXFRGADJTKP-UHFFFAOYSA-K |
SMILES | I[Lu](I)I |
Canonical SMILES | [I-].[I-].[I-].[Lu+3] |
Introduction
Physical and Chemical Properties
Lutetium(III) iodide possesses distinctive physical and chemical properties that determine its behavior and applications. Table 1 summarizes the key physical properties of this compound.
Table 1: Physical Properties of Lutetium(III) Iodide
Elemental Composition
The elemental analysis of lutetium(III) iodide reveals its precise composition by weight percentage, which is important for quality control and analytical procedures.
Table 2: Elemental Composition of Lutetium(III) Iodide
Synthesis and Preparation Methods
Several established methods exist for the synthesis of lutetium(III) iodide, each with specific advantages depending on the intended purity and scale of production. The primary synthesis routes are detailed below.
Direct Reaction with Iodine
The most straightforward method involves the direct reaction of lutetium metal with elemental iodine, represented by the following chemical equation:
2Lu + 3I₂ → 2LuI₃
This direct synthesis method is often preferred in laboratory settings due to its relative simplicity and efficiency.
Reaction with Mercury(II) Iodide
Another established synthetic route involves reacting metallic lutetium with mercury(II) iodide in vacuum conditions at an elevated temperature of approximately 500°C:
2Lu + 3HgI₂ → 2LuI₃ + 3Hg
An advantage of this method is that the elemental mercury generated during the reaction can be removed by distillation, yielding purified lutetium(III) iodide .
Preparation from Hydrated Form
Lutetium(III) iodide hydrate, which crystallizes from solution, can be converted to the anhydrous form by heating it with ammonium iodide . This dehydration method is useful when starting with hydrated lutetium compounds.
Crystal Structure and Molecular Geometry
The crystal structure of lutetium(III) iodide follows the bismuth(III) iodide-type arrangement . In this structure, lutetium atoms adopt an octahedral geometry, with each lutetium ion coordinated to six iodide ions . This 6-coordinate octahedral arrangement is characteristic of many lanthanide halides and influences the compound's physical and chemical properties.
X-ray crystallographic studies have revealed that in certain complexes, lutetium(III) iodide can exhibit auto-ionization, forming ionic structures with well-separated cations and anions. For instance, reaction products can include structures with [LuI₂(solvent)n]⁺ cations and [I]⁻ anions . This behavior is attributed to the relatively small ionic radius of the lutetium center and can significantly impact the compound's reactivity and coordination chemistry.
Chemical Reactions and Behavior
Lutetium(III) iodide displays several characteristic chemical reactions that are important for its applications and handling.
Hydrolysis
Due to its hygroscopic nature, lutetium(III) iodide readily reacts with moisture in the air to form hydrates. Further exposure to water can lead to hydrolysis, potentially resulting in the formation of lutetium hydroxide compounds and hydroiodic acid .
Oxidation
At elevated temperatures, lutetium(III) iodide can form the corresponding oxide iodide through partial oxidation . This reaction is relevant when considering the thermal stability of the compound in various applications.
Coordination Chemistry
Lutetium(III) iodide can form various coordination complexes with Lewis bases such as alcohols, ethers, and nitrogen-containing compounds. For example, reactions with 2-propanol or pyridine can lead to the formation of complexes like LuI₃(HO-iPr)₄ or [LuI₂(py)₅][I] . These coordination reactions are significant for understanding the behavior of lutetium(III) iodide in different solvent systems and its potential applications in catalysis.
Applications
Lutetium(III) iodide has found several important applications across different technological fields, with its most notable uses being in radiation detection and scintillation technologies.
Radiation Detection and Scintillation
When doped with cerium, lutetium(III) iodide (LuI₃:Ce) functions as an effective scintillation material for radiation detection . The compound exhibits several properties that make it valuable for this application:
-
High effective atomic number (Z = 59.7), which provides excellent stopping power for radiation
-
High density (5.6 g/cm³), enhancing radiation absorption efficiency
-
When properly doped with cerium, it produces high light yields in response to radiation
These properties make lutetium(III) iodide particularly suitable for use in positron emission tomography (PET) scanners and other radiation detection equipment .
Neutron and Gamma Radiation Detection
Lutetium(III) iodide can be used in combination with yttrium iodide and gadolinium iodide in LuI₃-YI₃-GdI₃ scintillator systems designed to detect both neutron and gamma radiation . This multi-element approach enhances the detection capabilities of the scintillator system.
Research Applications
Beyond its applications in radiation detection, lutetium(III) iodide serves as an important compound in various research areas:
-
Laboratory research in coordination chemistry
-
Materials science investigations
-
Development of optical sensors
Cerium-Doped Lutetium Iodide (LuI₃:Ce)
Cerium-doped lutetium iodide (LuI₃:Ce) represents one of the most important derivatives of lutetium(III) iodide, with specialized applications in high-speed imaging and radiation detection. This material combines the favorable properties of the lutetium iodide host lattice with the luminescent properties of cerium dopant.
Table 3: Properties of Cerium-Doped Lutetium Iodide (LuI₃:Ce)
Fabrication and Engineering
LuI₃:Ce scintillators can be fabricated in various forms:
-
Typical size of 40 mm diameter, with availability up to 4" × 4" dimensions
-
Tailorable resolution, efficiency, and physical dimensions based on application requirements
The concentration of cerium dopant can be adjusted to optimize the emission peak for specific applications, providing versatility in detector design .
- mass of a compound required to prepare a solution of known volume and concentration
- volume of solution required to dissolve a compound of known mass to a desired concentration
- concentration of a solution resulting from a known mass of compound in a specific volume