1,3-Dimethoxypropane
CAS No.: 17081-21-9
Cat. No.: VC21021124
Molecular Formula: C5H12O2
Molecular Weight: 104.15 g/mol
* For research use only. Not for human or veterinary use.
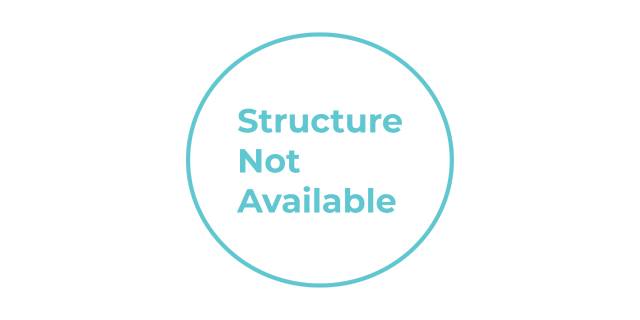
Specification
CAS No. | 17081-21-9 |
---|---|
Molecular Formula | C5H12O2 |
Molecular Weight | 104.15 g/mol |
IUPAC Name | 1,3-dimethoxypropane |
Standard InChI | InChI=1S/C5H12O2/c1-6-4-3-5-7-2/h3-5H2,1-2H3 |
Standard InChI Key | UUAMLBIYJDPGFU-UHFFFAOYSA-N |
SMILES | COCCCOC |
Canonical SMILES | COCCCOC |
Introduction
Chemical Identity and Structural Characteristics
Molecular Structure and Identification
1,3-Dimethoxypropane is characterized by a three-carbon propane chain with methoxy groups (-OCH₃) attached to the first and third carbon atoms. The compound has a molecular formula of C₅H₁₂O₂ and a molecular weight of 104.15 g/mol . This symmetric dialkyl ether is also known by several alternative names including trimethylene glycol dimethyl ether .
The structural representation of 1,3-dimethoxypropane can be expressed in SMILES notation as COCCCOC, with the InChI key UUAMLBIYJDPGFU-UHFFFAOYSA-N . This structural arrangement, with oxygen atoms positioned at the terminal positions of the carbon chain, contributes significantly to the compound's chemical behavior and physical properties.
Physical Properties
1,3-Dimethoxypropane exhibits physical properties that make it valuable for various applications, particularly as a solvent in organic synthesis. The key physical properties are summarized in Table 1:
Table 1: Physical Properties of 1,3-Dimethoxypropane
Property | Value |
---|---|
Molecular Weight | 104.15 g/mol |
Density | 0.8±0.1 g/cm³ |
Boiling Point | 102.2±8.0 °C at 760 mmHg |
Melting Point | -82 °C |
Flash Point | 7.8±11.8 °C |
These properties are derived from experimental measurements and computational studies . The relatively low boiling point indicates high volatility, while the low melting point suggests minimal intermolecular forces in the solid state. The density is comparable to other similar ethers, reflecting its molecular structure and intermolecular interactions.
Synthesis Methods and Production
Laboratory Synthesis from 3-Methoxy-1-propanol
One established method for synthesizing 1,3-dimethoxypropane involves the conversion of 3-methoxy-1-propanol under specific reaction conditions. This approach utilizes sulfuric acid as a catalyst at elevated temperatures, as demonstrated in comparative studies documented by ChemicalBook .
The procedure typically involves:
-
Charging a stainless steel autoclave with 0.10 g of sulfuric acid, 5.00 g of deionized water, and 1.00 g of 3-methoxy-l-propanol
-
Replacing air with nitrogen through pressurization and depressurization cycles
-
Heating the mixture to 220°C while stirring
-
Maintaining the reaction for 10 hours
-
Cooling to room temperature and analyzing the product by gas chromatography
This method has been shown to produce 1,3-dimethoxypropane without detectable carbonyl byproducts such as acrolein, 3-allyloxy-1-propionaldehyde, or 3-hydroxy-1-propionaldehyde .
Synthesis from 1,3-Propanediol
A more common synthetic route involves the acid-catalyzed etherification of 1,3-propanediol with methanol. This reaction proceeds according to the following equation:
HOCH₂CH₂CH₂OH + 2CH₃OH → CH₃OCH₂CH₂CH₂OCH₃ + 2H₂O
The reaction typically utilizes acid catalysts such as sulfuric acid or p-toluenesulfonic acid under reflux conditions. This approach represents a direct and efficient method for industrial-scale production, as it employs readily available starting materials and straightforward reaction conditions.
Industrial Production Considerations
For large-scale industrial production, continuous flow reactors are often employed to optimize reaction conditions and improve yields. The industrial process generally follows these key steps:
-
Mixing 1,3-propanediol and methanol in appropriate stoichiometric ratios
-
Addition of an acid catalyst to facilitate the reaction
-
Heating under reflux conditions to drive the reaction forward
-
Removing water through azeotropic distillation to shift equilibrium toward product formation
-
Purification through fractional distillation to obtain high-purity 1,3-dimethoxypropane
These industrial methods are designed to maximize yield while minimizing waste and energy consumption, making the production process economically viable and environmentally sustainable.
Conformational Analysis and Theoretical Studies
Quantum Chemical Investigations
Extensive theoretical studies have been conducted to understand the conformational behavior of 1,3-dimethoxypropane. Research published in the Journal of Physical Chemistry employed ab initio electronic structure calculations to determine the conformational energies and geometries of significant conformers .
These studies revealed that the predicted NMR vicinal coupling constants in 1,3-dimethoxypropane, using conformational populations determined from quantum chemical energies calculated at the MP2 level with a D95+(2df,p) basis set, demonstrate excellent agreement with experimental gas phase values . This validation confirms the accuracy of the computational approaches used to study this molecule's behavior.
Applications in Battery Technology
Lithium-Metal Battery Electrolytes
One of the most significant recent applications of 1,3-dimethoxypropane is in advanced lithium-metal battery electrolytes. Traditional ether electrolytes typically exhibit good reduction stability but suffer from inferior oxidation stability (below 4.0 V vs. Li/Li+), limiting their compatibility with high-voltage cathodes .
Research published in 2023 demonstrated that 1,3-dimethoxypropane forms a unique six-membered chelating complex with lithium ions (Li+), providing stronger solvating ability that effectively suppresses undesired oxidation side reactions . This distinctive coordination configuration plays a crucial role in enhancing electrolyte oxidation stability.
Enhanced Cathode Interface Formation
The improved performance of 1,3-dimethoxypropane in battery applications also stems from its ability to promote favorable interfaces with cathode materials. Studies have shown that the hydrogen transfer reaction between 1,3-dimethoxypropane and salt anions induces a significant enrichment of lithium fluoride (LiF) on the cathode surface, reaching a total atomic ratio of 76.7% .
As a result of these beneficial properties, electrolytes based on 1,3-dimethoxypropane demonstrate remarkably stable cycling of nickel-rich cathodes under high voltage conditions of 4.7 V, achieving 87% capacity retention after 150 cycles . This performance represents a significant advancement for high-energy-density battery development.
Chemical Reactivity and Transformation Pathways
Characteristic Reactions
1,3-Dimethoxypropane participates in various chemical reactions typical of ethers, while also exhibiting unique reactivity patterns influenced by its specific molecular structure. The compound can function as:
-
A nucleophile in substitution reactions
-
A substrate for oxidation to form corresponding aldehydes or carboxylic acids
-
A material for reduction to produce alcohols
-
A Lewis base in coordination chemistry, particularly with metal ions
The oxygen atoms in the methoxy groups serve as electron donors, enabling the molecule to participate in coordination chemistry with various metal ions and Lewis acids. This property is particularly valuable in catalytic applications and in the development of specialized reagents for organic synthesis.
Spectroscopic Characterization
Characterization of 1,3-dimethoxypropane and monitoring of its reactions can be accomplished through various spectroscopic methods. Mass spectrometry data for the compound shows characteristic fragment ions with m/z values of 45 (relative intensity 999), 72 (672), 41 (124), 43 (45), and 73 (45) .
These spectroscopic fingerprints provide essential tools for tracking the compound's presence and transformations in complex reaction mixtures, facilitating both research and quality control in industrial applications.
Specialized Research Applications
Polymer Science Investigations
1,3-Dimethoxypropane has been utilized as a model compound for understanding the behavior of more complex polymeric systems. Research on poly(oxytrimethylene) (POM3) has employed the conformational properties of 1,3-dimethoxypropane to develop accurate rotational isomeric state models .
These studies have revealed that the characteristic ratio of the polymer is in fair agreement with experimental observations, although temperature coefficients and mean-square dipole moment values show some discrepancies compared to other polyether models . These differences have been attributed to significant contributions from polymer-solvent interactions that depend on local chain conformations and the temperature dependence of condensed phase effects.
Structural Chemistry Applications
The structural properties of 1,3-dimethoxypropane make it valuable for investigating fundamental aspects of molecular behavior. Its relatively simple structure, combined with the conformational flexibility of the propane backbone, provides an ideal model system for studying intramolecular interactions and their effects on molecular properties .
Research utilizing molecular mechanics (MM3) and advanced conformational search techniques has employed 1,3-dimethoxypropane to understand the energetic ordering of molecular conformations and the factors that influence conformational stability . These insights contribute to our fundamental understanding of structural chemistry and help predict the behavior of more complex molecular systems.
- mass of a compound required to prepare a solution of known volume and concentration
- volume of solution required to dissolve a compound of known mass to a desired concentration
- concentration of a solution resulting from a known mass of compound in a specific volume