Ruthenium, trichloronitrosyl-
CAS No.: 18902-42-6
Cat. No.: VC21054514
Molecular Formula: Cl3NORu-
Molecular Weight: 237.4 g/mol
* For research use only. Not for human or veterinary use.
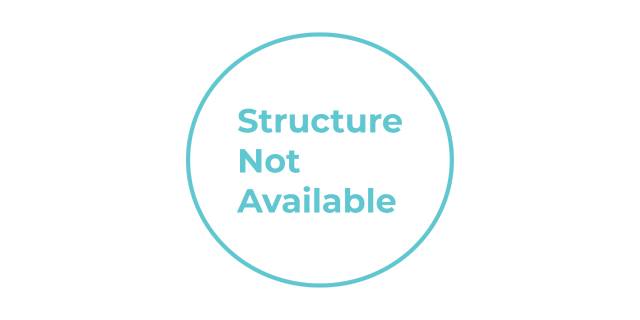
Specification
CAS No. | 18902-42-6 |
---|---|
Molecular Formula | Cl3NORu- |
Molecular Weight | 237.4 g/mol |
IUPAC Name | nitroxyl anion;trichlororuthenium |
Standard InChI | InChI=1S/3ClH.NO.Ru/c;;;1-2;/h3*1H;;/q;;;-1;+3/p-3 |
Standard InChI Key | DDZBQMRLQLDFJT-UHFFFAOYSA-K |
SMILES | [N-]=O.Cl[Ru](Cl)Cl |
Canonical SMILES | [N-]=O.Cl[Ru](Cl)Cl |
Introduction
Chemical Structure and Properties
Molecular Composition and Structure
Ruthenium trichloronitrosyl in its basic form has the molecular formula Cl₃NORu. The hydrated form, Ruthenium(III) Nitrosyl Chloride Hydrate, has the formula Cl₃NORu·xH₂O, where x represents the variable number of water molecules in the crystal structure . The molecular weight of the anhydrous form is approximately 237.44 g/mol, making it a relatively small coordination complex .
The structure features a central ruthenium atom coordinated to three chloride ligands and one nitrosyl group. The Ru-NO bond is typically linear, with bond angles close to 180°, reflecting the strong π-bonding characteristics of the nitrosyl ligand. This linear arrangement is consistent with the bonding model that involves significant metal-to-ligand π-backbonding.
Physical Properties
Ruthenium(III) Nitrosyl Chloride Hydrate appears as a dark red to dark brown powder with distinct physical properties that make it suitable for various applications . The compound has:
Property | Value |
---|---|
Appearance | Dark red to dark brown powder |
Melting Point | Approximately 500°C |
Density | 3100 kg/m³ |
Solubility | Limited solubility in water, more soluble in polar organic solvents |
Molecular Weight | 237.44 g/mol (anhydrous) |
The compound exhibits high thermal stability, making it suitable for applications involving elevated temperatures, such as in glass, optic, and ceramic applications .
Electronic Structure and Bonding
The electronic structure of ruthenium trichloronitrosyl involves significant π-backbonding between the metal center and the nitrosyl ligand. This interaction contributes to the stability of the complex and influences its reactivity patterns. The ruthenium center typically exists in the +3 oxidation state in this compound, though the strong π-acceptor character of the nitrosyl ligand can make the effective oxidation state somewhat ambiguous.
Spectroscopic studies of related ruthenium nitrosyl complexes have provided insights into the nature of the Ru-NO bond. In similar compounds, the Ru-N bond lengths typically range from 1.7 to 1.8 Å, with N-O distances around 1.1 to 1.2 Å, reflecting the multiple-bond character of both these connections .
Synthesis and Preparation Methods
Standard Synthetic Routes
Ruthenium trichloronitrosyl compounds are typically synthesized from ruthenium trichloride as the starting material. One common approach involves the reaction of ruthenium trichloride with nitric oxide or nitrite salts under controlled conditions. The hydrated form, Ruthenium(III) Nitrosyl Chloride Hydrate, can be prepared from the reaction of ruthenium trichloride trihydrate with appropriate nitrosylating agents.
The synthesis typically yields a product that is thermally stable and can be handled using standard laboratory techniques, though precautions should be taken due to its corrosive nature.
Alternative Preparation Methods
More complex derivatives of ruthenium trichloronitrosyl can be prepared by introducing additional ligands. For example, Trichloronitrosylbis(triphenylphosphine)ruthenium is synthesized by introducing triphenylphosphine ligands to the coordination sphere . This demonstrates the versatility of the ruthenium trichloronitrosyl core as a building block for more elaborate structures.
The reaction conditions, including temperature, solvent choice, and reaction time, significantly impact the yield and purity of the desired product. Careful control of these parameters is essential for successful synthesis.
Derivatives and Related Complexes
Phosphine Complexes
Complex | Ru-N Bond Length (Å) | N-O Bond Length (Å) | Ru-N-O Angle (°) |
---|---|---|---|
Ruthenium Trichloronitrosyl | ~1.8 | ~1.1 | ~178 |
Technetium Nitrosyl (for comparison) | 1.816 | 0.946 | 178.4 |
Photoactivatable Ru Complexes | ~2.03-2.11 | Not specified | Not specified |
Note: The data for technetium nitrosyl complexes is provided for comparison purposes, as they share similar structural features with ruthenium nitrosyl complexes .
Applications in Chemistry and Materials Science
Catalytic Applications
Ruthenium trichloronitrosyl and its derivatives have significant applications in catalysis. The compound serves as a precursor to various catalytically active ruthenium species, particularly in reactions involving:
-
Oxidation processes
-
Carbon-carbon bond formation
-
Hydrogenation reactions
-
Transfer hydrogenation processes
The unique electronic properties of the ruthenium-nitrosyl bond contribute to the catalytic activity of these complexes, making them valuable tools in synthetic chemistry.
Materials Science Applications
In materials science, Ruthenium(III) Nitrosyl Chloride Hydrate serves as a ruthenium source for various applications, including:
-
Glass manufacturing
-
Optical materials
-
Ceramic applications
-
Thin film deposition
The compound's thermal stability makes it particularly suitable for high-temperature applications, while its specific electronic properties contribute to the functional characteristics of the resulting materials .
Precursor for Complex Synthesis
One of the most important applications of ruthenium trichloronitrosyl is as a precursor for the synthesis of more complex ruthenium compounds. For example, Dichlorotris(triphenylphosphine)ruthenium(II) can be synthesized from ruthenium trichloride (which is related to ruthenium trichloronitrosyl) through reaction with triphenylphosphine . These derived complexes often find applications in homogeneous catalysis and materials chemistry.
Characterization and Analytical Methods
Spectroscopic Characterization
Several spectroscopic techniques prove valuable for characterizing ruthenium trichloronitrosyl and its derivatives:
-
Infrared Spectroscopy: The N-O stretching frequency in ruthenium nitrosyl complexes typically appears in the range of 1800-1900 cm⁻¹, providing a diagnostic tool for identifying these compounds.
-
Nuclear Magnetic Resonance (NMR): For derivatives containing phosphine ligands, ³¹P NMR spectroscopy offers insights into the coordination environment around the ruthenium center.
-
UV-Visible Spectroscopy: Ruthenium complexes often display characteristic absorption bands in the visible region, which can be used for identification and purity assessment.
X-ray Crystallography
X-ray crystallography has been instrumental in determining the precise structural parameters of ruthenium nitrosyl complexes. Studies of related compounds have revealed important structural features such as:
-
The nearly linear Ru-N-O arrangement (typical bond angles around 174-178°)
-
The relatively short Ru-N bond lengths (typically 1.7-1.8 Å)
-
The coordination geometry around the ruthenium center
These structural insights help explain the reactivity patterns and spectroscopic properties of these compounds .
Electrochemical Methods
Electrochemical techniques such as cyclic voltammetry provide valuable information about the redox properties of ruthenium nitrosyl complexes. These studies reveal the accessible oxidation states of the ruthenium center and the influence of the nitrosyl ligand on the electronic structure of the complex.
Current Research and Future Directions
Recent Advances
Recent research on ruthenium nitrosyl complexes has focused on understanding the electronic structure of these compounds and exploring their potential in new applications. Photoactivatable ruthenium complexes containing nitrosyl ligands have attracted particular attention for their potential in photoactivated drug delivery and catalysis .
Studies examining the role of ruthenium nitrosyl complexes in trinuclear and tetranuclear clusters have expanded our understanding of how these compounds interact with other metal centers, opening new possibilities for multimetallic catalysts .
Future Research Directions
Several promising research directions for ruthenium trichloronitrosyl and related compounds include:
-
Development of new catalytic systems for sustainable chemistry applications
-
Exploration of photochemical properties for energy conversion and storage
-
Investigation of biological applications, particularly in the context of NO release
-
Design of novel materials with specific electronic and optical properties
The versatility of ruthenium nitrosyl chemistry suggests that these compounds will continue to play an important role in various fields of chemistry and materials science.
- mass of a compound required to prepare a solution of known volume and concentration
- volume of solution required to dissolve a compound of known mass to a desired concentration
- concentration of a solution resulting from a known mass of compound in a specific volume