Divanillin, also known as 5, 5'-bivanillin or dehydrodivanillin, belongs to the class of organic compounds known as biphenyls and derivatives. These are organic compounds containing to benzene rings linked together by a C-C bond. Divanillin is considered to be a practically insoluble (in water) and relatively neutral molecule. Within the cell, divanillin is primarily located in the membrane (predicted from logP). Divanillin has a fruity and vanilla taste.
Dehydrodivanillin
CAS No.: 2092-49-1
Cat. No.: VC21061544
Molecular Formula: C16H14O6
Molecular Weight: 302.28 g/mol
* For research use only. Not for human or veterinary use.
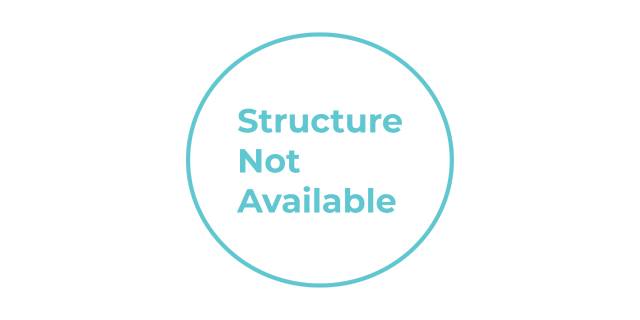
Specification
CAS No. | 2092-49-1 |
---|---|
Molecular Formula | C16H14O6 |
Molecular Weight | 302.28 g/mol |
IUPAC Name | 3-(5-formyl-2-hydroxy-3-methoxyphenyl)-4-hydroxy-5-methoxybenzaldehyde |
Standard InChI | InChI=1S/C16H14O6/c1-21-13-5-9(7-17)3-11(15(13)19)12-4-10(8-18)6-14(22-2)16(12)20/h3-8,19-20H,1-2H3 |
Standard InChI Key | NSTQUZVZBUTVPY-UHFFFAOYSA-N |
SMILES | COC1=CC(=CC(=C1O)C2=C(C(=CC(=C2)C=O)OC)O)C=O |
Canonical SMILES | COC1=CC(=CC(=C1O)C2=C(C(=CC(=C2)C=O)OC)O)C=O |
Introduction
Chemical Structure and Properties
Molecular Structure and Identification
Dehydrodivanillin (6,6'-dihydroxy-5,5'-dimethoxy-[1,1'-biphenyl]-3,3'-dicarbaldehyde) is characterized by a dimeric structure consisting of two benzene rings linked by a carbon-carbon bond, with hydroxyl and methoxy functional groups strategically positioned around the aromatic rings. The compound features two aldehyde groups that contribute significantly to its reactivity and potential applications in various chemical syntheses. Its systematic IUPAC name identifies the specific arrangement of functional groups that define its molecular architecture.
The molecular formula of dehydrodivanillin is C16H14O6 with a molecular weight of 302.29 g/mol, though some literature sources report slight variations in these parameters. The compound is identified by CAS number 2092-49-1, which serves as a unique identifier in chemical databases and repositories for scientific research.
Table 1: Key Structural and Physical Properties of Dehydrodivanillin
Property | Description |
---|---|
Chemical Name | 6,6'-dihydroxy-5,5'-dimethoxy-[1,1'-biphenyl]-3,3'-dicarbaldehyde |
Molecular Formula | C16H14O6 |
Molecular Weight | 302.29 g/mol |
CAS Number | 2092-49-1 |
Appearance | Crystalline solid |
Structure | Symmetrical dimer of vanillin |
Spectroscopic Characteristics
Spectroscopic analysis provides crucial information about the structural features of dehydrodivanillin. Nuclear Magnetic Resonance (NMR) spectroscopy reveals distinct signal patterns that confirm its chemical structure. In 1H NMR analysis, the signal at 9.7 ppm corresponds to the two aldehyde protons, while signals at 7.5 and 7.2 ppm are attributed to the aromatic protons. The methoxy groups produce a characteristic signal at 3.8 ppm .
The 13C NMR spectrum further validates the structure with signals at 191.2 ppm (carbonyl carbon), 150.4 and 148.16 ppm (oxygenated aromatic carbons), 128.2, 127.8, and 124.6 ppm (aromatic carbons), 109.2 ppm (aromatic carbon), and 56.0 ppm (methoxy carbon) . These spectroscopic data provide essential confirmation of the compound's structural integrity and purity.
Infrared (IR) spectroscopy of dehydrodivanillin shows characteristic absorption bands at 3220 cm-1 (OH stretching), 1676 cm-1 (C=O stretching), 1587 cm-1 (aromatic C=C stretching), and several other bands at 1413, 1245, 1127, and 750 cm-1, which correspond to various functional group vibrations . These spectroscopic fingerprints are invaluable for identification and quality assessment of the compound.
Natural Occurrence and Biosynthesis
Sources in Nature
Dehydrodivanillin occurs naturally in trace amounts in vanilla pods, contributing to the complex flavor profile of natural vanilla. The limited natural availability of this compound has necessitated the development of efficient synthetic methods for its production on a larger scale. Additionally, dehydrodivanillin has been identified as a product of lignin degradation in plants, linking it to natural metabolic processes related to plant cell wall components.
Biological Significance
In biological systems, dehydrodivanillin is known to interact with lignin-degrading microorganisms such as the actinomycete Streptomyces viridosporus T7A, which readily metabolizes this compound. This interaction suggests a role for dehydrodivanillin in natural lignin degradation pathways. Under strictly anaerobic conditions at 37°C, the compound has been found to be biodegradable by microflora from cow rumen fluid, indicating its integration into natural biochemical cycles.
The biological significance of dehydrodivanillin extends beyond its role in lignin degradation. Its structural features, particularly the phenolic functional groups, suggest potential bioactivity that may contribute to plant defense mechanisms or other physiological processes, although these aspects require further investigation to be fully understood.
Synthesis Methods
Enzymatic Approaches
Enzymatic synthesis has emerged as the preferred method for producing dehydrodivanillin due to its efficiency, selectivity, and environmentally friendly nature. A co-substrate independent dimerization of vanillin, conducted in a co-solvent system to improve the solubility of vanillin, has been developed using various fungal laccases .
Laccase-Mediated Synthesis
Fungal laccases from several sources have demonstrated effectiveness in catalyzing the formation of dehydrodivanillin:
-
Meripilus giganteus laccase produces the compound with high selectivity
-
Agaricus bisporus laccase shows good catalytic activity
-
Funalia trogii laccase (high-redox-potential) achieves exceptional yields exceeding 95%
-
Trametes versicolor laccase produces the compound in high yield (85%) and purity
The mechanism of laccase-catalyzed synthesis involves oxidative coupling of vanillin molecules. The kinetically preferred reaction product, 5,5'-dehydrodivanillin, rapidly reaches saturation and precipitates in situ, thus shifting the reaction equilibrium to favor product formation. This characteristic precipitation facilitates simple isolation of the product through filtration, enhancing the practical applicability of this synthesis approach .
Peroxidase-Catalyzed Production
Alternative enzymatic approaches involve peroxidase enzymes supplied with hydrogen peroxide:
-
Peroxidase from Marasmius scorodonius (MsP2) catalyzes the reaction with moderate efficiency
-
Horseradish peroxidase (HRP) produces dehydrodivanillin with yields of approximately 18%
While peroxidase-catalyzed methods are effective, they generally yield lower product amounts compared to laccase-mediated approaches, making the latter more attractive for larger-scale production.
Process Optimization and Scale-up
The synthesis of dehydrodivanillin has been optimized to enhance yield and facilitate potential scale-up. Using Trametes versicolor laccase, researchers have demonstrated that the dimer formed in the reaction precipitates spontaneously and can be recovered by simple filtration. The filtrate can then be reused by adding fresh vanillin and oxygen to restart the synthesis process, creating an efficient, potentially continuous production system .
Table 2: Comparison of Enzymatic Synthesis Methods for Dehydrodivanillin
Enzyme Source | Type | Yield (%) | Reaction Conditions | Recovery Method |
---|---|---|---|---|
Funalia trogii | Laccase | >95 | Co-solvent system | Precipitation/filtration |
Trametes versicolor | Laccase | 85 | Room temperature | Precipitation/filtration |
Horseradish | Peroxidase | 18 | With hydrogen peroxide | Extraction |
The development of efficient enzymatic synthesis methods represents a significant advancement in green chemistry approaches to producing value-added compounds from renewable resources. These methods align with principles of sustainability and bioeconomy, offering environmentally friendly alternatives to traditional chemical synthesis routes.
Molecular Interactions and Reactivity
Chemical Reactivity Patterns
Dehydrodivanillin exhibits characteristic reactivity patterns associated with its functional groups. The presence of aldehyde, hydroxyl, and methoxy groups on the aromatic rings contributes to its versatile chemical behavior. The aldehyde groups are particularly reactive, participating in various condensation reactions, while the hydroxyl groups facilitate hydrogen bonding and can undergo etherification reactions .
The compound can undergo several important chemical transformations:
-
Oxidation of the aldehyde groups to carboxylic acids
-
Reduction to corresponding alcohols
-
Condensation reactions with amines to form imines
-
Etherification of hydroxyl groups, as demonstrated in the synthesis of divanillin ethyl hexylated (DVEH)
These reactivity patterns are exploited in various synthetic applications, particularly in polymer chemistry where dehydrodivanillin serves as a valuable building block.
Interaction with Biological Systems
The interaction of dehydrodivanillin with biological systems primarily involves its metabolism by lignin-degrading organisms. As noted earlier, the lignin-degrading actinomycete Streptomyces viridosporus T7A readily catabolizes this compound. The biological degradation pathways likely involve oxidative processes that cleave the dimer into smaller metabolites, although the specific enzymatic mechanisms require further elucidation.
The potential bioactivity of dehydrodivanillin, including possible antioxidant properties associated with its phenolic structure, represents an area for future research. Understanding these interactions could reveal new applications in fields such as pharmacology or agricultural science.
Applications and Technological Relevance
Food Science Applications
Polymer | Co-monomer | Molar Mass (kg·mol-1) | Electronic Gap (eV) | Fluorescence Range (nm) |
---|---|---|---|---|
P1A | Benzene-1,4-diamine | ~10 | 2.66-2.85 | 400-600 |
P2 | 2,7-Diaminocarbazole | ~10 | 2.66-2.85 | 400-600 |
Sustainable Materials Development
The utilization of dehydrodivanillin in polymer synthesis represents an important step toward developing sustainable, biobased materials that can potentially replace petroleum-derived counterparts. By leveraging this lignin-derived compound, researchers are contributing to the broader effort of establishing circular bioeconomy approaches in materials science .
The lignin origin of dehydrodivanillin connects it to the concept of biorefinery, where multiple value-added products are derived from renewable biomass. This connection enhances its relevance in the context of sustainable development and green chemistry principles.
Research Frontiers and Future Directions
Emerging Research Areas
Current research on dehydrodivanillin spans multiple disciplines, reflecting its versatile nature and potential applications:
-
Development of more efficient and scalable synthesis methods
-
Exploration of novel polymerization pathways and polymer architectures
-
Investigation of potential bioactivities and pharmaceutical applications
-
Computational studies to understand structure-property relationships in dehydrodivanillin-based materials
Time-dependent density functional theory (TD-DFT) calculations have been employed to understand the electronic properties of divanillin-based polymers, corroborating experimental findings regarding their optical and electronic characteristics . Such computational approaches complement experimental work and accelerate the development of new applications.
Challenges and Opportunities
Despite significant progress, several challenges remain in dehydrodivanillin research:
-
Scaling up enzymatic synthesis methods for industrial-scale production
-
Optimizing polymer properties for specific applications
-
Exploring the full spectrum of potential applications in various fields
-
Integrating dehydrodivanillin production into biorefinery concepts
These challenges represent opportunities for interdisciplinary collaboration and innovation. Addressing them could lead to expanded applications and enhanced value creation from this renewable chemical platform.
- mass of a compound required to prepare a solution of known volume and concentration
- volume of solution required to dissolve a compound of known mass to a desired concentration
- concentration of a solution resulting from a known mass of compound in a specific volume