Glycidyl hexanoate
CAS No.: 17526-74-8
Cat. No.: VC21112047
Molecular Formula: C9H16O3
Molecular Weight: 172.22 g/mol
* For research use only. Not for human or veterinary use.
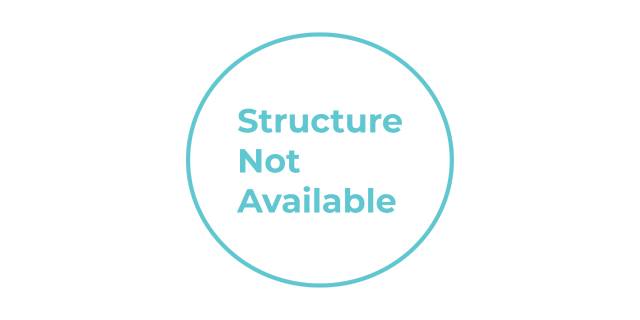
Specification
CAS No. | 17526-74-8 |
---|---|
Molecular Formula | C9H16O3 |
Molecular Weight | 172.22 g/mol |
IUPAC Name | oxiran-2-ylmethyl hexanoate |
Standard InChI | InChI=1S/C9H16O3/c1-2-3-4-5-9(10)12-7-8-6-11-8/h8H,2-7H2,1H3 |
Standard InChI Key | LYJNHHFSQGRJFP-UHFFFAOYSA-N |
SMILES | CCCCCC(=O)OCC1CO1 |
Canonical SMILES | CCCCCC(=O)OCC1CO1 |
Introduction
Chemical Structure and Properties
Molecular Structure and Identification
Glycidyl hexanoate features a characteristic epoxide ring (oxirane) connected to a hexanoate chain through an ester linkage. This structure can be represented through various chemical identifiers:
Property | Value |
---|---|
IUPAC Name | oxiran-2-ylmethyl hexanoate |
Molecular Formula | C9H16O3 |
InChI | InChI=1S/C9H16O3/c1-2-3-4-5-9(10)12-7-8-6-11-8/h8H,2-7H2,1H3 |
InChI Key | LYJNHHFSQGRJFP-UHFFFAOYSA-N |
Canonical SMILES | CCCCCC(=O)OCC1CO1 |
CAS Number | 17526-74-8 |
Molecular Weight | 172.22 g/mol |
The compound possesses a unique structural arrangement that contributes to its chemical behavior and applications.
Physical and Chemical Properties
Glycidyl hexanoate exists as a colorless liquid at room temperature. Its most distinctive chemical feature is the epoxide functional group, which consists of a three-membered ring containing an oxygen atom. This strained ring structure contributes significantly to the compound's reactivity, particularly in ring-opening reactions with nucleophiles.
The ester linkage provides another site for potential reactions, particularly hydrolysis and transesterification. The six-carbon alkyl chain imparts a degree of hydrophobicity to the molecule, affecting its solubility characteristics and interactions with other molecules.
Synthesis Methods
Laboratory Synthesis Pathways
The primary method for synthesizing glycidyl hexanoate involves the esterification of hexanoic acid with glycidol. This reaction typically requires catalysis to proceed efficiently:
-
Esterification Reaction: Hexanoic acid reacts with glycidol in the presence of acid catalysts such as sulfuric acid or p-toluenesulfonic acid.
-
Reaction Conditions: The process is typically conducted under reflux conditions to drive the reaction to completion.
-
Purification: The resulting product is usually purified through distillation to remove impurities and unreacted starting materials.
The general reaction can be represented as:
Hexanoic acid + Glycidol → Glycidyl hexanoate + Water
Chemical Reactivity
General Reactivity Profile
The reactivity of glycidyl hexanoate stems primarily from its epoxide group, which readily undergoes ring-opening reactions with various nucleophiles. The three-membered epoxide ring experiences significant ring strain, making it susceptible to nucleophilic attack. Additionally, the ester group provides another site for potential chemical transformations.
Oxidation Reactions
Glycidyl hexanoate can undergo oxidation reactions, particularly at the epoxide moiety:
-
Reagents: Hydrogen peroxide, peracids, and other oxidizing agents
-
Conditions: Typically mild conditions are employed to maintain selectivity
-
Products: Diols or other oxygenated derivatives depending on reaction conditions
-
Mechanism: Nucleophilic attack on the epoxide followed by ring-opening
Reduction Reactions
The ester functionality in glycidyl hexanoate can be reduced:
-
Reagents: Lithium aluminum hydride, sodium borohydride
-
Conditions: Controlled temperature and stoichiometry
-
Products: Reduction can yield hexanoic alcohol or glycidyl alcohol, depending on the selectivity of the reducing agent
-
Applications: These reduced products can serve as intermediates for further synthetic transformations
Nucleophilic Substitution Reactions
The epoxide ring readily undergoes nucleophilic substitution reactions:
-
Reagents: Amines, thiols, alcohols, and other nucleophiles
-
Conditions: Basic or neutral conditions typically favor these transformations
-
Products: Ring-opened products with the nucleophile incorporated into the structure
-
Importance: These reactions form the basis for many applications of glycidyl hexanoate in polymer chemistry and organic synthesis
Reaction Mechanisms
The primary mechanism for many reactions of glycidyl hexanoate involves nucleophilic attack at the epoxide carbon atoms. Due to the ring strain, the epoxide readily opens to form more stable products. This mechanism is particularly important in cross-linking reactions, where glycidyl hexanoate can form bonds with multiple nucleophilic groups, creating networked structures.
Applications in Research and Industry
Role in Organic Synthesis
Glycidyl hexanoate serves as a versatile building block in organic synthesis:
-
Intermediate Compound: Its reactive epoxide group makes it valuable for introducing specific functional groups into molecules
-
Diversity-Oriented Synthesis: The compound can be used to create diverse molecular scaffolds through selective reactions at either the epoxide or ester moiety
-
Functional Group Transformations: The epoxide serves as a masked diol, allowing for regioselective introduction of hydroxyl groups
Comparison with Structurally Related Compounds
Structural Analogues and Their Properties
Several glycidyl esters share structural similarities with glycidyl hexanoate but differ in specific features that affect their properties and applications:
Compound | Key Structural Difference | Effect on Properties |
---|---|---|
Glycidyl acetate | Shorter alkyl chain (2 carbon) | Increased hydrophilicity, different solubility profile |
Glycidyl neodecanoate | Branched 10-carbon chain | Enhanced steric hindrance, different reactivity pattern |
Glycidyl methacrylate | Contains unsaturated methacrylate group | Polymerizable through the methacrylate group |
These structural differences result in distinct reactivity profiles and applications for each compound .
Structure-Activity Relationships
The chain length and structure of the alkyl portion of glycidyl esters significantly influence their physical properties and applications:
-
Chain Length Effects: The six-carbon chain of glycidyl hexanoate provides intermediate hydrophobicity compared to shorter-chain and longer-chain analogues
-
Branching Effects: Compared to branched analogues like glycidyl neodecanoate, glycidyl hexanoate has a more linear structure, affecting its steric properties
-
Functional Group Interactions: The relative positioning of the epoxide and ester groups creates unique reactivity profiles for each glycidyl ester
For instance, acrylated glycidyl neodecanoate (AGN), which combines glycidyl neodecanoate with acrylic functionality, demonstrates how structural modifications can significantly alter compound properties and applications. AGN contains hydroxyl functionality and a hydrophobic branched structure that enhances compatibility with certain crosslinkers in coating applications .
Analytical Identification and Characterization
Spectroscopic Methods
Several spectroscopic techniques can be employed for the identification and characterization of glycidyl hexanoate:
-
Nuclear Magnetic Resonance (NMR) Spectroscopy: Provides structural information through characteristic chemical shifts
-
Proton NMR (1H NMR): Shows signals for the epoxide protons and the aliphatic chain
-
Carbon NMR (13C NMR): Reveals the carbon skeleton structure
-
Phosphorus NMR (31P NMR): Can be used to track reactions involving phosphorus reagents, with similar epoxide reactions showing characteristic signals around 146.3 ppm
-
-
Infrared (IR) Spectroscopy: Identifies functional groups through characteristic absorption bands
-
Epoxide ring: Typically shows bands around 1250 and 850 cm-1
-
Ester carbonyl: Strong absorption around 1730-1750 cm-1
-
-
Mass Spectrometry: Provides molecular weight confirmation and fragmentation patterns
-
Molecular ion: Corresponds to the molecular weight of 172.22 g/mol
-
Fragmentation pattern: Characteristic fragments including loss of the epoxide group
-
Chromatographic Analysis
Chromatographic techniques offer additional methods for identification and purity assessment:
-
Gas Chromatography (GC): Suitable for volatile compounds like glycidyl hexanoate
-
High-Performance Liquid Chromatography (HPLC): Provides separation and quantification
-
Thin-Layer Chromatography (TLC): Useful for reaction monitoring and purity checks
- mass of a compound required to prepare a solution of known volume and concentration
- volume of solution required to dissolve a compound of known mass to a desired concentration
- concentration of a solution resulting from a known mass of compound in a specific volume