Chlorophyll
CAS No.: 479-61-8
Cat. No.: VC21318266
Molecular Formula: C55H73MgN4O5+
Molecular Weight: 894.5 g/mol
* For research use only. Not for human or veterinary use.
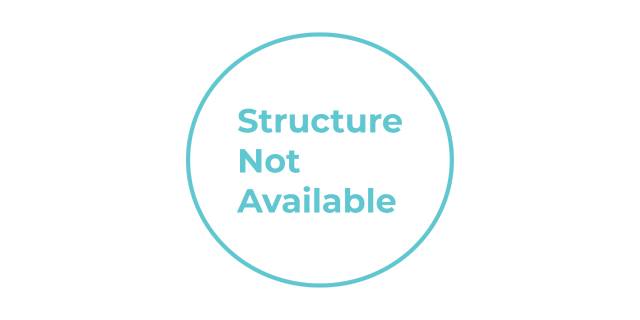
CAS No. | 479-61-8 |
---|---|
Molecular Formula | C55H73MgN4O5+ |
Molecular Weight | 894.5 g/mol |
IUPAC Name | magnesium;(3R,21S,22S)-16-ethenyl-11-ethyl-3-methoxycarbonyl-12,17,21,26-tetramethyl-22-[3-oxo-3-[(E,7R,11R)-3,7,11,15-tetramethylhexadec-2-enoxy]propyl]-7,23,24,25-tetrazahexacyclo[18.2.1.15,8.110,13.115,18.02,6]hexacosa-1,4,6,8(26),9,11,13(25),14,16,18(24),19-undecaen-4-olate |
Standard InChI | InChI=1S/C55H74N4O5.Mg/c1-13-39-35(8)42-28-44-37(10)41(24-25-48(60)64-27-26-34(7)23-17-22-33(6)21-16-20-32(5)19-15-18-31(3)4)52(58-44)50-51(55(62)63-12)54(61)49-38(11)45(59-53(49)50)30-47-40(14-2)36(9)43(57-47)29-46(39)56-42;/h13,26,28-33,37,41,51,58,61H,1,14-25,27H2,2-12H3;/q;+2/p-1/b34-26+,44-28?,46-29?,47-30?,52-50?;/t32-,33-,37+,41+,51-;/m1./s1 |
Standard InChI Key | BAPACHZCGHDRCQ-VZWGJMBDSA-M |
Isomeric SMILES | CCC1=C(C2=NC1=CC3=C(C4=C([C@@H](C(=C5[C@H]([C@@H](C(=CC6=NC(=C2)C(=C6C)C=C)N5)C)CCC(=O)OC/C=C(\C)/CCC[C@H](C)CCC[C@H](C)CCCC(C)C)C4=N3)C(=O)OC)[O-])C)C.[Mg+2] |
SMILES | CCC1=C(C2=NC1=CC3=C(C4=C([N-]3)C(=C5C(C(C(=N5)C=C6C(=C(C(=C2)[N-]6)C=C)C)C)CCC(=O)OCC=C(C)CCCC(C)CCCC(C)CCCC(C)C)C(C4=O)C(=O)OC)C)C.[Mg+2] |
Canonical SMILES | CCC1=C(C2=NC1=CC3=C(C4=C(C(C(=C5C(C(C(=CC6=NC(=C2)C(=C6C)C=C)N5)C)CCC(=O)OCC=C(C)CCCC(C)CCCC(C)CCCC(C)C)C4=N3)C(=O)OC)[O-])C)C.[Mg+2] |
Melting Point | 117 - 120 °C |
Historical Discovery and Research
Evolution of Chlorophyll Research
Following Willstätter's foundational discoveries, chlorophyll research expanded dramatically throughout the 20th century. Scientists developed increasingly sophisticated techniques to examine chlorophyll's three-dimensional structure, elucidate its biosynthetic pathways, and determine its precise mechanisms in photosynthetic systems. Modern analytical methods now allow researchers to detect and quantify chlorophyll with exceptional precision, enabling its use as a biomarker in environmental monitoring and ecological research.
Molecular Structure and Properties
Basic Structural Components
All chlorophyll molecules share a fundamental architecture consisting of:
-
A porphyrin ring (specifically a chlorin structure) with four nitrogen atoms
-
A central magnesium atom coordinated by these nitrogen atoms
-
A long hydrophobic phytol tail that anchors the molecule in photosynthetic membranes
The porphyrin ring contains an extensive system of conjugated double bonds that allows chlorophyll to absorb specific wavelengths of visible light, predominantly in the blue and red regions of the spectrum. This accounts for plants' characteristic green appearance, as green light is primarily reflected rather than absorbed.
Types of Chlorophyll
Multiple forms of chlorophyll exist across different photosynthetic organisms, each with subtle structural variations that affect their spectral properties and ecological roles.
Chlorophyll a and b
Chlorophyll a (C₅₅H₇₂MgN₄O₅) serves as the primary photosynthetic pigment in all oxygen-producing organisms. It functions directly in the reaction centers where the conversion of light energy to chemical energy occurs.
Chlorophyll b (C₅₅H₇₀MgN₄O₆) has a molecular weight of approximately 907.5 g/mol and differs from chlorophyll a by having a formyl group (-CHO) instead of a methyl group (-CH₃) at a specific position on the chlorin ring . This structural difference shifts its absorption spectrum, allowing it to capture wavelengths of light that chlorophyll a cannot efficiently absorb, thereby expanding the range of light energy available for photosynthesis.
Other Chlorophyll Variants
Additional chlorophyll forms include chlorophyll c (found in various algae), chlorophyll d and f (present in some cyanobacteria), and bacteriochlorophylls (in photosynthetic bacteria). These specialized variants have evolved to harvest light in specific ecological niches, particularly in aquatic environments where light availability and spectral quality differ from terrestrial settings.
Property | Chlorophyll a | Chlorophyll b |
---|---|---|
Molecular Formula | C₅₅H₇₂MgN₄O₅ | C₅₅H₇₀MgN₄O₆ |
Molecular Weight | 893.5 g/mol | 907.5 g/mol |
Color in Solution | Blue-green | Yellow-green |
Structural Difference | Methyl group | Formyl group |
Primary Role | Reaction center pigment | Accessory light-harvesting pigment |
Maximum Absorption (nm) | 430 and 662 | 453 and 642 |
Distribution | All photosynthetic plants | Higher plants and green algae |
Biological Function in Photosynthesis
Organization in Photosynthetic Apparatus
Chlorophyll molecules are housed within chloroplasts, specialized organelles found in plant cells, particularly concentrated in leaves and other green tissues. Within chloroplasts, chlorophyll is embedded in the thylakoid membranes, organized into sophisticated protein-pigment complexes called photosystems .
Two distinct photosystems operate in plants and algae:
-
Photosystem I (PSI)
-
Photosystem II (PSII)
Each photosystem contains numerous chlorophyll molecules arranged around a specialized reaction center. This arrangement allows for efficient light energy capture and transfer to the reaction center, where photochemical reactions initiate the conversion of light energy to chemical energy .
Light Absorption and Energy Transfer
When chlorophyll absorbs a photon of light, the energy excites an electron to a higher energy state. This excitation energy is not used directly but instead transfers through a series of neighboring pigment molecules until reaching the reaction center. This energy transfer process, known as resonance energy transfer, occurs with remarkable efficiency in less than a nanosecond.
The photosystems contain "antenna complexes" where numerous chlorophyll molecules (along with other accessory pigments) work collectively to capture light and funnel the energy to reaction centers. Photons are captured as discrete packets of energy and then this energy is channeled through the photosynthetic apparatus with minimal loss, demonstrating the exceptional efficiency of natural light-harvesting systems.
At the reaction center, the energy initiates electron transport chains that ultimately produce ATP (adenosine triphosphate) and NADPH (nicotinamide adenine dinucleotide phosphate), the energy currency and reducing power needed for carbon fixation in the Calvin cycle.
Measurement and Analysis Methods
Extraction Techniques
Accurate chlorophyll quantification begins with effective extraction from biological samples. Common solvents include:
-
Acetone (typically 90%)
-
Ethanol (typically 90-95%)
-
Methanol
Research comparing extraction methodologies has demonstrated that different solvents can yield varying results. Multiple studies have indicated that ethanol often functions as a more efficient extractant of chlorophyll a than acetone . This methodological difference represents an important consideration when comparing results across studies or laboratories.
The typical extraction process involves:
-
Sample collection and preparation (filtering water samples or homogenizing plant tissue)
-
Addition of the appropriate extraction solvent
-
Tissue disruption to release chlorophyll
-
Incubation (often in darkness and at low temperatures)
-
Centrifugation to separate the chlorophyll-containing solution
-
Analysis using appropriate quantification methods
Quantification Methodologies
Several techniques exist for measuring chlorophyll concentration:
Spectrophotometry
The most widely used method involves measuring light absorbance at specific wavelengths characteristic of chlorophyll (approximately 664-665 nm for chlorophyll a and 647-649 nm for chlorophyll b). Mathematical equations then convert these absorbance values to chlorophyll concentrations.
High-Performance Liquid Chromatography (HPLC)
HPLC offers superior specificity by separating chlorophyll from other pigments before quantification. This technique is particularly valuable when analyzing samples with complex pigment compositions or when measuring chlorophyll degradation products.
Fluorometry
This approach exploits chlorophyll's natural fluorescence properties, providing high sensitivity particularly useful for in situ measurements in aquatic environments.
Methodological Considerations
An inter-laboratory comparison study examining chlorophyll a analysis from periphyton samples revealed important insights into methodological factors affecting measurement accuracy. While chlorophyll estimates from six different laboratories showed strong correlations (R > 0.97), systematic biases existed between different analytical approaches .
The study found that laboratories using 90% ethanol as the extractant demonstrated relatively modest discrepancies among themselves, while greater differences appeared when comparing results between ethanol and acetone extraction methods . This highlights the critical importance of standardized protocols for reliable chlorophyll quantification, particularly in regulatory monitoring or comparative research applications.
Environmental Applications and Monitoring
Water Quality Assessment
A comprehensive compilation of chlorophyll a data from United States water bodies spanning 18 years (2005-2022) represents an unprecedented resource, including nearly 84,000 sampling sites and over 1,374,000 individual pigment measurements . This extensive dataset supports various applications, including:
-
Calibration of harmful algal bloom prediction models
-
Validation of remote sensing approaches
-
Examination of relationships between chlorophyll and environmental drivers
-
Evaluation of long-term water quality trends
The dataset reveals significant spatial and temporal variations in chlorophyll concentrations across American water bodies, with marked differences in sampling frequency, distribution, and methodologies between states . Such large-scale monitoring efforts provide crucial information for detecting ecosystem changes resulting from factors including nutrient pollution, climate change, and land use alterations.
Ecological Research Applications
Beyond water quality assessment, chlorophyll measurements provide valuable ecological information across diverse research contexts. In terrestrial ecosystems, chlorophyll content serves as an indicator of plant health, photosynthetic capacity, and response to environmental stressors.
In specialized symbiotic organisms such as lichens, chlorophyll content reveals important insights into physiological adaptations. Research on the lichen Cetraria aculeata examined chlorophyll content, chlorophyll a/b ratios, and algal cell densities across populations from different geographical locations, documenting physiological adaptations to diverse environmental conditions .
Remote sensing of chlorophyll has revolutionized large-scale ecological monitoring, enabling researchers to track global patterns of primary productivity, detect algal blooms, and assess ecosystem responses to environmental changes across unprecedented spatial and temporal scales.
Current Research Directions
Analytical Method Refinement
Recent research has focused on improving analytical methods for chlorophyll detection and quantification. The 2024 publication documenting the comprehensive U.S. chlorophyll a dataset represents a significant advancement in data harmonization, addressing challenges including standardization of reporting units, pigment type classifications, and quality control procedures .
Inter-laboratory comparison studies have highlighted the need for greater methodological consistency. Research examining chlorophyll analysis across six laboratories found that while measurements showed strong correlations, systematic biases existed depending on extraction methods employed . Such findings emphasize the importance of standardized protocols, particularly for regulatory monitoring and long-term research programs.
Environmental Monitoring Applications
The application of chlorophyll measurements to environmental monitoring continues to expand, particularly in aquatic ecosystems where chlorophyll serves as an indicator for:
-
Harmful algal bloom detection and prediction
-
Eutrophication assessment
-
Climate change impacts on aquatic primary production
-
Effectiveness of water quality management interventions
The harmonized 18-year chlorophyll dataset for U.S. water bodies provides an unprecedented resource for addressing local to national-scale water quality concerns . Such comprehensive datasets enable researchers to develop more sophisticated models relating chlorophyll dynamics to environmental drivers and human impacts.
Molecular and Genetic Investigations
At the molecular level, research continues to advance understanding of chlorophyll biosynthesis, regulation, and degradation pathways. These investigations have implications for:
-
Agricultural applications (improving crop photosynthetic efficiency)
-
Biotechnology (engineering photosynthetic systems for biofuel production)
-
Environmental adaptation (understanding how plants respond to changing conditions)
Future Perspectives
Integration with Remote Sensing
The future of chlorophyll research increasingly involves integration with remote sensing technologies. Satellite-based chlorophyll monitoring enables global-scale observations of primary productivity patterns and detection of large algal bloom events. Ground-truthing these remote observations with accurate laboratory measurements remains a critical challenge, underscoring the importance of standardized analytical methods.
Climate Change Implications
As climate change alters temperature regimes, precipitation patterns, and atmospheric carbon dioxide levels, understanding chlorophyll dynamics becomes increasingly important for predicting ecosystem responses. Changes in chlorophyll concentrations may serve as early indicators of shifting ecological conditions in both aquatic and terrestrial systems.
Technological Innovations
Emerging technologies including advanced spectroscopic methods, automated sampling platforms, and real-time monitoring systems promise to revolutionize chlorophyll measurement capabilities. These innovations will enable more comprehensive spatial and temporal coverage while reducing analytical costs and labor requirements.
Mass Molarity Calculator
- mass of a compound required to prepare a solution of known volume and concentration
- volume of solution required to dissolve a compound of known mass to a desired concentration
- concentration of a solution resulting from a known mass of compound in a specific volume