Patulin is a natural product found in Trichoderma virens, Amesia atrobrunnea, and other organisms with data available.
Patulin is found in pomes. Mycotoxin, found as a contaminant of foods, e.g. apple juice. Sometimes detd. in apple juice Patulin is a mycotoxin produced by a variety of molds, particularly Aspergillus and Penicillium. It is commonly found in rotting apples, and the amount of patulin in apple products is generally viewed as a measure of the quality of the apples used in production. It is not a particularly potent toxin, but a number of studies have shown that it is genotoxic, which has led to some theories that it may be a carcinogen, though animal studies have remained inconclusive. Patulin is also an antibiotic. Several countries have instituted patulin restrictions in apple products. The World Health Organization recommends a maximum concentration of 50 ug/L in apple juice. Patulin has been shown to exhibit apoptotic and antibiotic functions (A7849, A7850). Patulin belongs to the family of Pyrans. These are compounds containing a pyran ring, which is a six-member heterocyclic, non-aromatic ring with five carbon atoms, one oxygen atom and two ring double bonds.
4-Hydroxy-4H-furo(3,2-c)pyran-2(6H)-one. A mycotoxin produced by several species of Aspergillus and Penicillium. It is found in unfermented apple and grape juice and field crops. It has antibiotic properties and has been shown to be carcinogenic and mutagenic and causes chromosome damage in biological systems.
Patulin
CAS No.: 149-29-1
Cat. No.: VC21334771
Molecular Formula: C7H6O4
Molecular Weight: 154.12 g/mol
* For research use only. Not for human or veterinary use.
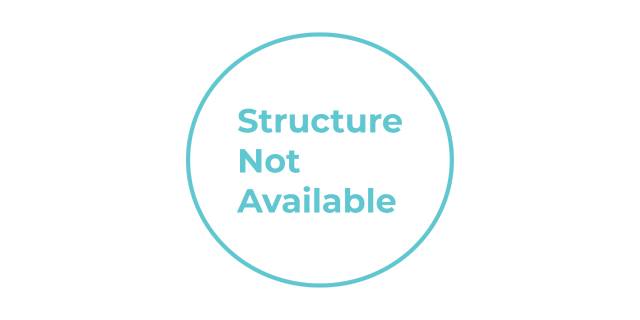
CAS No. | 149-29-1 |
---|---|
Molecular Formula | C7H6O4 |
Molecular Weight | 154.12 g/mol |
IUPAC Name | 4-hydroxy-4,6-dihydrofuro[3,2-c]pyran-2-one |
Standard InChI | InChI=1S/C7H6O4/c8-6-3-4-5(11-6)1-2-10-7(4)9/h1,3,7,9H,2H2 |
Standard InChI Key | ZRWPUFFVAOMMNM-UHFFFAOYSA-N |
SMILES | C1C=C2C(=CC(=O)O2)C(O1)O |
Canonical SMILES | C1C=C2C(=CC(=O)O2)C(O1)O |
Colorform | Compact prisms or thick plates from ether or chloroform White...crystalline solid CRYSTALS FROM BENZENE Colorless crystals, prisms, or plates from Et2O or trichloromethane |
Melting Point | 111.0 °C 111 °C |
Biosynthesis and Chemical Properties
Biosynthetic Pathway
The biosynthesis of patulin follows a complex polyketide pathway involving multiple enzymatic reactions. The process begins with the condensation of acetate units to form 6-methylsalicylic acid (6-MSA), which serves as a key intermediate. Subsequent transformations through a series of oxidative reactions, including decarboxylation and cyclization steps, yield the final patulin structure. This process is mediated by a complex of fungal enzymes that facilitate the conversion of precursors into the active mycotoxin .
Chemical Reactivity and Stability
Patulin demonstrates high reactivity toward sulfhydryl groups, which explains its ability to bind to and inhibit many enzymes containing these functional groups . This reactivity is particularly relevant when considering patulin's interactions with cysteine, where adduct formation has been observed. Interestingly, these patulin-cysteine adducts exhibit reduced toxicity compared to unmodified patulin in acute toxicity, teratogenicity, and mutagenicity studies .
Toxicological Profile
General Toxicity Mechanisms
Patulin's primary toxicity mechanism involves its strong affinity for sulfhydryl groups, enabling it to inhibit many enzymes essential for cellular functions . This mechanism explains the broad spectrum of toxicological effects observed in various biological systems. Studies have shown that patulin exposure induces oxidative stress in cells, as evidenced by decreased superoxide dismutase (SOD) and glutathione peroxidase activities, alongside increased malondialdehyde (MDA) content .
At the cellular level, patulin has been demonstrated to increase mitochondrial membrane permeability, leading to decreased ATP levels and accumulation of reactive oxygen species (ROS) . This mitochondrial dysfunction ultimately triggers apoptotic pathways, as evidenced by the release of cytochrome C and activation of the caspase enzyme cascade system .
Acute Toxicity
The acute toxicity of patulin varies across species, with rodents showing higher sensitivity compared to poultry. Oral LD50 values in rodents range between 29 and 55 mg/kg body weight, while poultry demonstrate an oral LD50 of approximately 170 mg/kg body weight . The toxicity of patulin increases significantly when administered via intravenous, intraperitoneal, or subcutaneous routes, being 3-6 times more toxic than oral administration .
Acute exposure to patulin produces a constellation of toxic effects, including:
-
Agitation and convulsions
-
Dyspnea
-
Pulmonary congestion and edema
-
Gastrointestinal effects including ulceration, hyperemia, and distension
In humans, reported acute symptoms primarily include gastrointestinal issues, nausea, and vomiting, though definitive evidence regarding chronic effects remains limited .
Chronic Toxicity Effects
Chronic exposure to patulin has been associated with a range of toxicological effects in experimental animals. These include:
-
Congestion and edema of blood vessels and tissues
-
Potential carcinogenicity concerns, as evidenced by sarcoma formation following large-dose injections in animals
-
Teratogenicity
-
Liver, spleen, and kidney damage
-
Lung and brain edema
While these effects have been well-documented in animal studies, conclusive evidence regarding the chronic effects in humans remains limited, highlighting a significant research gap in this area .
Cellular and Molecular Mechanisms of Toxicity
Recent research has elucidated the cellular and molecular mechanisms underlying patulin toxicity. A study using 293 T cells demonstrated that patulin induces cell injury in a time and concentration-dependent manner . The specific pathways involved include:
-
Induction of oxidative stress through decreased SOD and glutathione peroxidase activities
-
Mitochondrial dysfunction characterized by decreased ATP levels and increased ROS accumulation
-
Increased mitochondrial membrane permeability leading to cytochrome C release
-
Activation of the caspase enzyme cascade, with caspase 3 identified as the most critical component, exhibiting strong correlations with caspase 8 and caspase 9 activities
This activation of the mitochondrial apoptosis pathway appears to be a central mechanism in patulin-induced cellular toxicity.
Patulin Contamination in Food
Common Food Sources
While patulin is primarily associated with apples and apple-derived products, its presence has been detected in a variety of food commodities. Table 1 summarizes the common food sources of patulin contamination.
Table 1: Common Food Sources of Patulin Contamination
Food Category | Specific Products |
---|---|
Fruits | Apples, pears, figs, tomatoes |
Vegetables | Bell peppers |
Grains | Wheat, rice, corn |
Dairy | Some cheeses |
Nuts | Peanuts, pecans, hazelnuts |
Apple-based products, particularly juices and purees, represent the most significant source of patulin exposure for consumers, which has led to the establishment of specific regulatory limits for these products .
Global Prevalence and Contamination Levels
Numerous surveys worldwide have documented patulin contamination in commercial food products, with some samples exceeding regulatory limits. A study in Tunisia examining apple and pear products found that 50% of samples were contaminated with patulin, with levels ranging from 2 μg/kg to as high as 889 μg/kg. Notably, 22% of the contaminated samples exceeded the European Union's regulatory limit . Similarly, research in Turkey discovered patulin contamination in dried figs at concentrations reaching 151 μg/kg .
Other studies have focused on detecting the presence of patulin-producing fungi in food products. Fungal species such as P. expansum, B. nivea, and A. terreus have been isolated from various cereals and fruits, while strains of patulin-producing fungi have been found in nuts including peanuts, pecans, and hazelnuts . The presence of these fungi raises concerns about potential post-processing production of patulin under favorable conditions.
Regulatory Standards
Given the potential health risks associated with patulin exposure, regulatory agencies worldwide have established maximum allowable levels in various food products. Table 2 outlines the current regulatory standards for patulin in different food categories.
Table 2: Regulatory Limits for Patulin in Food Products
Food Product | Maximum Allowable Level | Regulatory Authority |
---|---|---|
Apple juice | 50 μg/L | WHO, EU, FDA |
Solid apples | 50 μg/kg | WHO, EU, FDA |
Kids and baby apple-based foods | 10 μg/L | WHO, EU, FDA |
These limits serve as quality indicators for food products and guide monitoring efforts to ensure consumer safety . Despite these regulations, patulin continues to be detected in commercial products, sometimes exceeding established limits, highlighting the ongoing challenge of controlling this contaminant in the food supply .
Detection and Analytical Methods
Accurate and sensitive detection methods are essential for monitoring patulin levels in food products and ensuring compliance with regulatory standards. While the search results don't provide extensive details on analytical methods, it's worth noting that various techniques have been developed for patulin detection, including chromatographic methods such as HPLC (High-Performance Liquid Chromatography) coupled with different detection systems.
The detection of patulin serves as a quality indicator for raw apples and apple-based products, providing valuable information about potential fungal contamination and storage conditions . This application underscores the importance of reliable analytical methods for both regulatory compliance and quality control purposes.
Mitigation and Degradation
Thermal Processing Effects
Thermal processing represents a potential strategy for reducing patulin levels in food products. Research investigating the thermal stability and degradation kinetics of patulin has revealed temperature-dependent effects. In phosphoric-citric acid buffer solutions (pH 3.5), patulin degradation increases with higher temperatures and longer heating times .
A study examining temperatures ranging from 90 to 150°C demonstrated that degradation efficiency reached 24.50%, 37.97%, and 54.49% after 30 minutes at 90, 120, and 150°C, respectively. When the heating time was extended to 90 minutes, the degradation efficiencies increased to 42.12%, 78.94%, and 100% at the same respective temperatures . These findings indicate that high-temperature processing, particularly for extended durations, can significantly reduce patulin levels in acidic food products.
Chemical Degradation Methods
The addition of certain compounds, particularly those containing sulfhydryl groups, can enhance patulin degradation. Research has shown that cysteine effectively promotes patulin degradation under acidic conditions at elevated temperatures. In a study using phosphoric-citric acid buffer (pH 3.5) with added cysteine (30 μmol/L), patulin degradation was significantly enhanced compared to thermal treatment alone .
The degradation kinetics of patulin in the presence of cysteine followed first-order and Weibull models, with activation energies of 43.89 kJ/mol, lower than the 61.74 kJ/mol observed for thermal degradation alone . This reduced activation energy indicates that cysteine facilitates patulin degradation, potentially through several mechanisms:
-
Nucleophilic addition reactions between cysteine's active sites (-SH, -NH2, and -COOH groups) and patulin
-
A combination of thermal degradation and nucleophilic addition, where patulin first degrades to intermediates (3-keto-5-hydroxypentanal and glyoxylic acid) that subsequently react with cysteine
-
Formation of hydrogen bonds and covalent bonds between patulin and cysteine in high-acid, high-temperature environments
These findings suggest potential strategies for reducing patulin levels in food processing through the strategic addition of sulfhydryl-containing compounds.
Human Health Risk Assessment
Human exposure to patulin occurs primarily through the consumption of contaminated food products, with apple juice and other apple-based foods representing the most significant sources. The health risks associated with patulin exposure depend on both the concentration in food and the consumption patterns of individuals, particularly vulnerable populations such as infants and young children .
The establishment of maximum allowable levels for patulin (50 μg/L in apple juice, 50 μg/kg in solid apples, and 10 μg/L in children's apple-based foods) reflects risk assessment considerations aimed at protecting consumer health . These limits acknowledge the potentially greater vulnerability of infants and young children to patulin's toxic effects, as evidenced by the lower permissible level for children's products.
While acute patulin exposure in humans has been associated with gastrointestinal symptoms including nausea and vomiting, the potential long-term effects of chronic low-level exposure remain an area of ongoing research and concern . The continued detection of patulin in commercial food products, sometimes exceeding regulatory limits, highlights the importance of rigorous monitoring and effective mitigation strategies to minimize consumer exposure and associated health risks.
Mass Molarity Calculator
- mass of a compound required to prepare a solution of known volume and concentration
- volume of solution required to dissolve a compound of known mass to a desired concentration
- concentration of a solution resulting from a known mass of compound in a specific volume