Squalamine is a natural product found in Squalus acanthias with data available.
Squalamine
CAS No.: 148717-90-2
Cat. No.: VC21338579
Molecular Formula: C34H65N3O5S
Molecular Weight: 628.0 g/mol
* For research use only. Not for human or veterinary use.
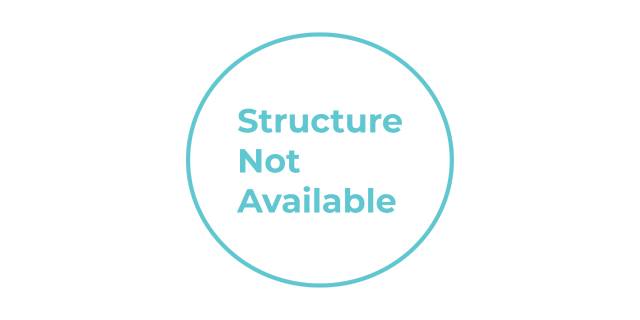
Specification
CAS No. | 148717-90-2 |
---|---|
Molecular Formula | C34H65N3O5S |
Molecular Weight | 628.0 g/mol |
IUPAC Name | [(3R,6R)-6-[(3S,5R,7R,8R,9S,10S,13R,14S,17R)-3-[3-(4-aminobutylamino)propylamino]-7-hydroxy-10,13-dimethyl-2,3,4,5,6,7,8,9,11,12,14,15,16,17-tetradecahydro-1H-cyclopenta[a]phenanthren-17-yl]-2-methylheptan-3-yl] hydrogen sulfate |
Standard InChI | InChI=1S/C34H65N3O5S/c1-23(2)31(42-43(39,40)41)12-9-24(3)27-10-11-28-32-29(14-16-34(27,28)5)33(4)15-13-26(21-25(33)22-30(32)38)37-20-8-19-36-18-7-6-17-35/h23-32,36-38H,6-22,35H2,1-5H3,(H,39,40,41)/t24-,25-,26+,27-,28+,29+,30-,31-,32+,33+,34-/m1/s1 |
Standard InChI Key | UIRKNQLZZXALBI-MSVGPLKSSA-N |
Isomeric SMILES | C[C@H](CC[C@H](C(C)C)OS(=O)(=O)O)[C@H]1CC[C@@H]2[C@@]1(CC[C@H]3[C@H]2[C@@H](C[C@@H]4[C@@]3(CC[C@@H](C4)NCCCNCCCCN)C)O)C |
SMILES | CC(C)C(CCC(C)C1CCC2C1(CCC3C2C(CC4C3(CCC(C4)NCCCNCCCCN)C)O)C)OS(=O)(=O)O |
Canonical SMILES | CC(C)C(CCC(C)C1CCC2C1(CCC3C2C(CC4C3(CCC(C4)NCCCNCCCCN)C)O)C)OS(=O)(=O)O |
Introduction
Origin and Discovery
Squalamine was initially isolated from the tissues of the dogfish shark (Squalus acanthias) and the sea lamprey (Petromyzon marinus) during a search for antimicrobial agents . This aminosterol compound was identified through natural product screening methods, though it can now be readily synthesized in laboratory settings . The discovery of squalamine emerged from broader research into bioactive compounds derived from marine organisms, which have historically yielded numerous valuable pharmaceutical candidates. The original isolation process focused on compounds with potential antimicrobial properties, but subsequent research revealed squalamine's diverse biological activities beyond this initial application domain.
Natural Sources
Squalamine occurs naturally in the liver of the dogfish shark and tissues of the sea lamprey, where it likely serves protective functions for these organisms . These marine species have evolved unique biochemical defense mechanisms, with squalamine potentially serving as part of their innate immune response against pathogens. The compound's presence in these evolutionarily ancient vertebrates suggests its biological role may be conserved across diverse species. The natural concentrations of squalamine in these organisms vary by tissue type and physiological conditions, with the liver typically containing the highest concentrations.
Synthetic Production
While initially isolated from natural sources, squalamine can now be chemically synthesized, facilitating its availability for research and potential therapeutic applications . The ability to synthetically produce squalamine has been crucial for advancing clinical research, as it removes dependency on harvesting from marine organisms. The synthetic pathway for squalamine production has been optimized to yield a pure compound identical to the naturally occurring version, enabling consistent quality for both research and potential therapeutic applications.
Chemical Structure and Properties
Squalamine is classified as a cationic amphipathic sterol, exhibiting unique physicochemical properties that underlie its biological activities . Its chemical structure consists of a sterol backbone with attached amino groups that confer its characteristic positive charge. This chemical architecture allows squalamine to interact with negatively charged biological membranes through electrostatic forces.
Molecular Characteristics
The aminosterol structure gives squalamine a positive charge density that enables unusual interactions with cell membranes . This positive charge density is nearly equal and opposite to the charge density of lipid components on the anionic cytoplasmic surface of typical eukaryotic membranes, resulting in exceptionally strong membrane binding . The amphipathic nature of squalamine, having both hydrophilic and hydrophobic regions, allows it to integrate into lipid bilayers and potentially disrupt membrane-dependent processes.
Electrostatic Properties
Squalamine's surface charge density facilitates strong binding to biological membranes through the maximal recovery of counterion entropy . This property enables squalamine to displace membrane-bound cationic proteins such as Rac1, a ρ-GTPase that plays a critical role in actin remodeling necessary for endocytosis . The displacement occurs because squalamine exhibits stronger electrostatic binding than many cellular proteins to charged bilayers, effectively doubling the counterion release that dominates the free-energy change upon binding .
Mechanism of Action
Squalamine exhibits multiple mechanisms of action across different biological systems, with its primary effects relating to membrane electrostatics and protein displacement.
Membrane Interactions
The principal mechanism underlying squalamine's biological effects involves its ability to alter the electrostatic potential of cellular membranes . Using synchrotron small-angle X-ray scattering, researchers have demonstrated that squalamine molecules can sandwich between the polar faces of packed bilayers at a spatial periodicity of 5.3 nm . This interaction results in sheets of bilayers with squalamine molecules distributed throughout the membrane structure. When incubated with membranes containing both Rac1 (a membrane-associated protein) and squalamine, diffraction patterns show that squalamine-membrane binding occurs at the expense of Rac1-membrane complexes, indicating squalamine's ability to displace membrane-associated proteins .
Protein Displacement
Molecular dynamics simulations have confirmed squalamine's ability to displace membrane-bound proteins. At concentrations sufficient for either Rac1 or squalamine to saturate the membrane, squalamine displaces approximately 56% of all Rac1 from the membrane . This displacement results from squalamine's stronger electrostatic binding to charged bilayers compared to many cellular proteins, effectively doubling the counterion release that dominates the free-energy change upon binding .
Antiangiogenic Effects
Squalamine's antiangiogenic properties appear to be mediated through inhibition of the sodium-hydrogen antiporter sodium-proton exchangers, specifically the NHE3 isoform . This inhibition causes a reduction in hydrogen ion efflux from endothelial cells, consequently reducing cellular proliferation . Studies have shown that squalamine selectively inhibits new blood vessel formation, with the greatest effects observed on newly emerging vessels and minimal impact on unstimulated endothelial cells . The compound blocks mitogen-induced proliferation and migration of endothelial cells without directly affecting the growth of tumor cells in culture .
Antiviral Properties
Squalamine has demonstrated broad-spectrum antiviral activity against both RNA and DNA enveloped viruses in vitro and in vivo studies .
Spectrum of Antiviral Activity
Laboratory studies have confirmed squalamine's effectiveness against various human viral pathogens . The compound's broad-spectrum activity represents a significant advantage over conventional antivirals that typically target specific viral enzymes or proteins and are therefore limited to a narrow range of viral species. Squalamine appears effective against both RNA and DNA enveloped viruses, suggesting a mechanism that impacts fundamental aspects of viral replication common across diverse viral families .
Proposed Antiviral Mechanism
Squalamine is thought to exert its antiviral effects by altering the infectivity of host tissues through perturbation of the electrostatic potential of cellular membranes . Upon entering a cell, squalamine disturbs the intracellular arrangement of membrane-associated proteins positioned by electrostatic forces, potentially including those of host or viral origin required for viral replication . This mechanism differs significantly from conventional antiviral compounds that directly target viral proteins.
Resistance Development
Due to its mechanism targeting host cell membranes rather than specific viral components, development of viral resistance to squalamine is hypothesized to be unlikely . This characteristic represents a significant advantage over traditional antivirals, which often encounter resistance development through viral mutations. The researchers suggest that by modifying the electrostatic interactions of cellular membranes, squalamine could influence diverse intracellular processes, making cells less capable of supporting viral replication without providing a clear evolutionary pathway for viruses to develop resistance .
Anticancer Applications
Squalamine has shown promise as an anticancer agent, particularly through its antiangiogenic properties and potential synergy with conventional chemotherapeutics.
Preclinical Evidence
Studies in tumor-bearing mice have demonstrated that squalamine inhibits angiogenesis and tumor growth in xenograft models of various cancers, including lung, breast, ovarian, and prostate cancer, as well as in brain and breast allograft tumor models in rats . Squalamine has also been shown to prevent lung metastases in the murine Lewis lung carcinoma model, both as a single agent and in combination with various chemotherapeutic agents . Notably, squalamine appears to have limited direct effects on primary tumor growth when administered alone but demonstrates enhanced antitumor responses when combined with cytotoxic agents .
Clinical Trial Results
A Phase I/IIA clinical trial evaluated squalamine in combination with paclitaxel and carboplatin in patients with non-small cell lung cancer (NSCLC) . The study included 45 patients (18 in the Phase I dose escalation arm and 27 in the Phase IIA arm) . The results are summarized in the table below:
Parameter | Data |
---|---|
Total patients | 45 |
Evaluable patients | 43 |
Total courses administered | 172 |
Median courses per patient | 3 |
Range of courses per patient | 1–6 |
Median patient age (years) | 60 |
Age range (years) | 41–84 |
Gender distribution | 22 male, 23 female |
Performance status 0 at entry | 9 (20%) |
In terms of clinical response, among the 35 evaluable patients who completed at least two cycles of treatment, 12 patients (34%) achieved partial responses, 8 patients (23%) had stable disease, and 15 patients (43%) experienced progressive disease . For all treated patients, the median survival was 10.0 months, with a 1-year survival rate of 40% . For patients treated at the maximum tolerated dose of squalamine (300 mg/m²/day), the median survival was 8.5 months with a 1-year survival rate of 33% .
Pharmacokinetics
The pharmacokinetic profile of squalamine has been characterized in clinical studies, providing valuable insights into its behavior in the human body.
Absorption and Distribution
Squalamine was administered as a continuous infusion over 5 days in the Phase I/IIA trial . The initial distribution phase was quite short, with a distribution half-life ranging from 0.94 to 1.74 hours . This rapid distribution phase suggests quick tissue uptake following administration. The pharmacokinetic study revealed linear pharmacokinetics over the four dose levels tested, with maximum plasma concentration (Cmax) ranging from 2.7 to 10.7 μg/ml and area under the curve (AUC) ranging from 348 to 1301 μg/ml∗h .
Metabolism and Elimination
Squalamine plasma clearance follows second-order elimination kinetics, with clearance rates ranging from 4.67 to 7.55 liters/hour . The terminal half-life of squalamine ranged from 16.7 to 203.6 hours, with one patient at the 100-mg/m²/day dose level exhibiting an unusually long terminal half-life attributed to a very high concentration observed during the terminal portion of the concentration-time curve . This outlier may have resulted from inadequate flushing of squalamine in the central line after completion of infusion and before blood sample collection .
Administration Methods
Squalamine has been administered through different routes in preclinical and clinical studies, with varying pharmacokinetic profiles and therapeutic outcomes.
Intravenous Administration
In clinical trials, squalamine has been administered as a continuous intravenous infusion over 5 days . This administration method allows for controlled delivery and consistent plasma levels of the compound. The intravenous route bypasses first-pass metabolism, enabling higher bioavailability compared to potential oral formulations.
Intraperitoneal vs. Subcutaneous Administration
Preclinical studies in BALB/c mice infected with murine cytomegalovirus (MCMV) compared intraperitoneal (i.p.) and subcutaneous (s.c.) administration routes of squalamine . The study found that i.p. dosing resulted in a rapid rise in blood and tissue levels, peaking within 1 hour of administration and generally achieving levels 10-fold higher than the s.c. route . In contrast, s.c. dosing resulted in a slow release of compound from the injection site, reaching peak blood and tissue concentrations within 5–8 hours .
The differential pharmacokinetic profiles of these administration routes translated to varying therapeutic efficacies. Administration of squalamine through the i.p. route proved most effective in the MCMV mouse model, resulting in undetectable viral titers in both the liver and spleen at day 14 post-infection . While s.c. dosing was also effective in reducing viral titers in these organs, it was less effective than the i.p. route . These findings highlight the importance of administration route in optimizing squalamine's therapeutic effects.
Future Perspectives
Squalamine represents a promising therapeutic candidate with potential applications across multiple disease domains.
- mass of a compound required to prepare a solution of known volume and concentration
- volume of solution required to dissolve a compound of known mass to a desired concentration
- concentration of a solution resulting from a known mass of compound in a specific volume