(4S,5S)-5-hydroxy-2-methyl-1,4,5,6-tetrahydropyrimidine-4-carboxylic acid is a natural product found in Streptomyces albidoflavus, Streptomyces coelicolor, and Phaseolus vulgaris with data available.
Hydroxyectoine
CAS No.: 165542-15-4
Cat. No.: VC21342472
Molecular Formula: C6H10N2O3
Molecular Weight: 158.16 g/mol
* For research use only. Not for human or veterinary use.
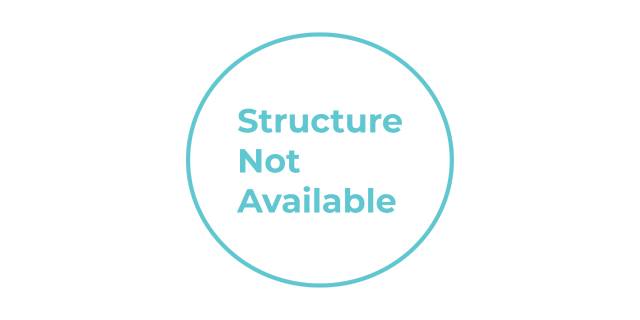
CAS No. | 165542-15-4 |
---|---|
Molecular Formula | C6H10N2O3 |
Molecular Weight | 158.16 g/mol |
IUPAC Name | (5S,6S)-5-hydroxy-2-methyl-1,4,5,6-tetrahydropyrimidine-6-carboxylic acid |
Standard InChI | InChI=1S/C6H10N2O3/c1-3-7-2-4(9)5(8-3)6(10)11/h4-5,9H,2H2,1H3,(H,7,8)(H,10,11)/t4-,5-/m0/s1 |
Standard InChI Key | KIIBBJKLKFTNQO-WHFBIAKZSA-N |
Isomeric SMILES | CC1=NC[C@@H]([C@H](N1)C(=O)O)O |
SMILES | CC1=NCC(C(N1)C(=O)O)O |
Canonical SMILES | CC1=NCC(C(N1)C(=O)O)O |
Chemical Structure and Properties
Hydroxyectoine ((4S,5S)-1,4,5,6-tetrahydro-5-hydroxy-2-methyl-4-pyrimidinecarboxylic acid) is the hydroxylated derivative of ectoine with the chemical formula C₆H₁₀N₂O₃ and a molecular weight of 158.16 g/mol . It features a tetrahydropyrimidine ring structure with a hydroxyl group at the 5-position that critically distinguishes it from ectoine . This single hydroxyl group significantly alters the compound's physical properties and protective capabilities.
Hydroxyectoine exists as a zwitterionic molecule at physiological pH, making it highly water-soluble (approximately 660 mg/ml) . It displays remarkable chemical and physical stability while remaining biologically inert, allowing it to accumulate at high intracellular concentrations (exceeding 1 M) without interfering with cellular functions . The additional hydroxyl group reduces molecular symmetry, preventing tight packing and creating space for optimized hydrogen bonding—properties crucial to its superior protective effects .
Property | Value |
---|---|
Chemical Formula | C₆H₁₀N₂O₃ |
Molecular Weight | 158.16 g/mol |
IUPAC Name | (4S,5S)-1,4,5,6-Tetrahydro-5-hydroxy-2-methyl-4-pyrimidinecarboxylic acid |
Synonyms | 5-Hydroxyectoine, β-Hydroxyectoine, Pyrostatin A |
Appearance | White solid |
Solubility in Water | ≈660 mg/ml |
CAS Number | 165542-15-4 |
Biosynthesis and Production
Hydroxyectoine is biosynthesized from ectoine through a hydroxylation reaction catalyzed by ectoine hydroxylase, a member of the non-heme-containing iron (II) and 2-oxoglutarate-dependent dioxygenase superfamily . This enzyme's activity depends on iron (II), molecular oxygen, and 2-oxoglutarate . The biosynthetic pathway involves:
-
Initial synthesis of ectoine from L-aspartate-β-semialdehyde through the EctABC pathway
-
Hydroxylation of ectoine at the C5 position by ectoine hydroxylase (EctD/ThpD)
The production of hydroxyectoine in bacteria is significantly influenced by environmental factors, particularly osmotic stress (high salinity) and thermal stress (high temperature) . Research with Halomonas elongata has demonstrated that the relative proportion of hydroxyectoine increases with both salinity and temperature, reaching approximately 70% at 45°C in high-salt conditions . Remarkably, a sudden temperature upshift to 50°C triggers a rapid increase in hydroxyectoine proportion to approximately 75% .
Commercial production methods include fermentation using halophilic bacteria and "bacterial milking" processes involving osmotic downshock to release accumulated hydroxyectoine . Heterologous production in engineered Escherichia coli has also been successful, achieving maximum productivity of 175 μmol/(g dry biomass·h) at 2% NaCl—exceeding previous records for ectoine production .
Temperature | NaCl Concentration | Relative Proportion of Hydroxyectoine |
---|---|---|
30°C | 8% NaCl | ≈17% |
30°C | 15% NaCl | ≈25% |
45°C | 15% NaCl | ≈70% |
50°C (upshock) | 15% NaCl | ≈75% |
Glass-Forming Properties
The most distinctive characteristic of hydroxyectoine is its exceptional glass-forming ability, which fundamentally explains its superior protective effects during desiccation . When dried from solution, hydroxyectoine forms clear, transparent solids without cracks, in stark contrast to ectoine, which tends to form crystalline inclusions .
This glass-forming property results from the additional hydroxyl group, which reduces molecular symmetry and prevents tight packing. The hydroxyl provides more possibilities for hydrogen bonding by increasing the number of donors, strengthening intermolecular interactions, and raising the energy barrier for molecular reorganization . Electron Spin Resonance (ESR) studies have revealed that dry hydroxyectoine creates a much more stable matrix for guest molecules than ectoine .
The glass transition temperature (Tg) of hydroxyectoine is approximately 87°C, significantly higher than ectoine (47°C) and even sucrose (65°C), though somewhat lower than trehalose (117°C) . This elevated Tg allows hydroxyectoine to maintain an amorphous glass state at higher temperatures, providing superior protection to biomolecules during desiccation at elevated temperatures .
Compatible Solute | Glass Transition Temperature (Tg) |
---|---|
Hydroxyectoine | ≈87°C |
Ectoine | ≈47°C |
Sucrose | ≈65°C |
Trehalose | ≈117°C |
Metabolic Analysis and Cellular Processing
Research has provided detailed insights into the metabolic processing of hydroxyectoine in bacterial cells. In Ruegeria pomeroyi DSS-3, hydroxyectoine is metabolized through a specific catabolic pathway, beginning with hydrolysis by the EutD enzyme to produce hydroxy-α-ADABA . This metabolite serves as a high-affinity ligand for the transcriptional regulator EnuR, with dissociation constants in the low micromolar range .
Targeted metabolic analysis of R. pomeroyi DSS-3 grown on hydroxyectoine as the sole carbon, energy, and nitrogen source revealed substantial amounts of hydroxy-α-ADABA in cellular extracts, along with smaller amounts of DABA and very low levels of hydroxy-DABA . These metabolites likely play important roles in regulating the expression of genes involved in hydroxyectoine catabolism.
Growth Substrate | α-ADABA | Hydroxy-α-ADABA | DABA | Hydroxy-DABA | γ-ADABA |
---|---|---|---|---|---|
Ectoine | High | Not detected | Present | Not detected | High |
5-Hydroxyectoine | Not detected | High | Present | Low | Very low |
Glucose + NH₄Cl | Not detected | Not detected | Not detected | Not detected | Not detected |
Protective Mechanisms
Hydroxyectoine exerts multiple protective effects on biological molecules and systems through several mechanisms:
Osmotic Protection
As a compatible solute, hydroxyectoine helps cells maintain osmotic balance in high-salinity environments by accumulating intracellularly without disrupting cellular functions . This osmotic protection allows halophilic bacteria to thrive in hypersaline environments.
Thermal Protection
Hydroxyectoine stabilizes proteins and cellular structures against thermal denaturation, which explains the increased proportion of hydroxyectoine production at higher temperatures in halophilic bacteria . This thermal protection is significantly superior to that provided by ectoine.
Desiccation Protection
The glass-forming properties of hydroxyectoine create a protective matrix around biomolecules during drying, preventing denaturation and aggregation . Experiments with H. elongata demonstrated that cells with 75% hydroxyectoine content had a survival rate of nearly 30% after harsh drying conditions (3 hours at 45°C and 10 mbar), compared to only 4.7% for cells with 17% hydroxyectoine .
Protein Stabilization
As a chemical chaperone, hydroxyectoine prevents protein aggregation and maintains enzymatic activity under stress conditions . In experiments with lactate dehydrogenase (LDH), hydroxyectoine preserved approximately 70% of enzyme activity after 2 hours of drying at 60°C, while ectoine provided no protection under the same conditions .
Protective Agent | Residual LDH Activity After 2h | Residual LDH Activity After 4h | Residual LDH Activity After 6h |
---|---|---|---|
Control (No Additive) | ≈5% | ≈2% | <1% |
Hydroxyectoine | ≈70% | ≈45% | ≈25% |
Ectoine | ≈5% | ≈2% | <1% |
Sucrose | ≈58% | ≈50% | ≈45% |
Trehalose | ≈83% | ≈75% | ≈65% |
Applications in Biotechnology and Biomedicine
Hydroxyectoine has diverse applications across biotechnology and biomedicine:
Biotechnological Applications
Hydroxyectoine serves as:
-
A protein protectant during freeze-thawing, lyophilization, and storage
-
A DNA protectant against degradation during storage and processing
The compound is particularly valuable for stabilizing antibodies during freeze-thaw cycles and preserving enzyme activity during lyophilization . For example, hydroxyectoine enables retention of 80% activity for lactate dehydrogenase and phosphofructokinase after lyophilization when added at 1 M concentration .
Biomedical Applications
In medicine, hydroxyectoine functions as:
Research has demonstrated its effectiveness as a novel anti-inflammatory compound with a special focus on inflammatory bowel disease and lung inflammation . It also protects manganese-depleted photosystem II against photoinhibition by acting as a source of electrons .
Comparison with Other Compatible Solutes
Hydroxyectoine offers several advantages over other compatible solutes:
Despite their structural similarity, hydroxyectoine exhibits superior protective effects compared to ectoine, particularly in desiccation protection and thermal stability . This superiority stems from hydroxyectoine's higher glass transition temperature and better glass-forming properties.
When compared with disaccharides like trehalose and sucrose—traditional benchmark glass-formers—hydroxyectoine's protective effects during desiccation are competitive. After 2 hours of drying at 60°C, hydroxyectoine preserved approximately 70% of LDH activity, compared to 58% for sucrose and 83% for trehalose .
Unlike ectoine, which fails to protect proteins during high-temperature desiccation (above its Tg of 47°C), hydroxyectoine remains effective at temperatures up to its Tg of 87°C . This makes hydroxyectoine particularly valuable for applications requiring both desiccation protection and thermal stability.
Current Research and Future Perspectives
Current research on hydroxyectoine focuses on:
Production optimization through strain engineering, bioprocess development, and metabolic pathway enhancement has made significant progress. Heterologous expression in E. coli has achieved productivity exceeding previous records without requiring additional pathway engineering .
Mechanism elucidation continues to provide deeper insights into hydroxyectoine's protective effects at the molecular level. ESR and FTIR studies have revealed important details about hydrogen bonding networks and molecular mobility in hydroxyectoine glasses .
Novel applications are being explored across various fields, including drug delivery, vaccine stabilization, and nanobiotechnology. The compound's ability to form stable glasses makes it promising for preserving bioactive molecules in extreme conditions.
Therapeutic development is investigating hydroxyectoine's potential for treating inflammatory conditions, respiratory diseases, and neurodegenerative disorders . Its natural origin and biocompatibility make it attractive for pharmaceutical applications.
Mass Molarity Calculator
- mass of a compound required to prepare a solution of known volume and concentration
- volume of solution required to dissolve a compound of known mass to a desired concentration
- concentration of a solution resulting from a known mass of compound in a specific volume