Pratensein is a natural product found in Cicer microphyllum, Dalbergia sissoo, and other organisms with data available.
See also: Trifolium pratense flower (part of).
Pratensein
CAS No.: 2284-31-3
Cat. No.: VC21345220
Molecular Formula: C16H12O6
Molecular Weight: 300.26 g/mol
* For research use only. Not for human or veterinary use.
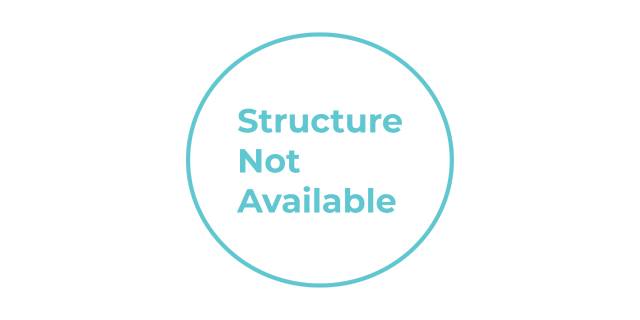
CAS No. | 2284-31-3 |
---|---|
Molecular Formula | C16H12O6 |
Molecular Weight | 300.26 g/mol |
IUPAC Name | 5,7-dihydroxy-3-(3-hydroxy-4-methoxyphenyl)chromen-4-one |
Standard InChI | InChI=1S/C16H12O6/c1-21-13-3-2-8(4-11(13)18)10-7-22-14-6-9(17)5-12(19)15(14)16(10)20/h2-7,17-19H,1H3 |
Standard InChI Key | FPIOBTBNRZPWJW-UHFFFAOYSA-N |
Canonical SMILES | COC1=C(C=C(C=C1)C2=COC3=CC(=CC(=C3C2=O)O)O)O |
Melting Point | 272 - 273 °C |
Chemical Properties and Structure
Identification and Nomenclature
Pratensein, identified by CAS Registry Number 2284-31-3, is chemically described as 5,7-dihydroxy-3-(3-hydroxy-4-methoxyphenyl)-4H-1-benzopyran-4-one . This compound is also known by several synonyms including 4′-methoxy-3′,5,7-trihydroxyisoflavone, 5,7,3′-trihydroxy-4′-methoxyisoflavone, and 3′-hydroxybiochanin A . The systematic naming reflects its structural characteristics as a modified isoflavone with specific substitution patterns that contribute to its biological activity profile.
Physical and Chemical Characteristics
Pratensein possesses distinct physical and chemical properties that are essential for understanding its behavior in biological systems. Table 1 summarizes these key characteristics:
The compound forms needle-like crystals when recrystallized from ethanol . These physical properties are important considerations for its extraction, purification, and formulation for potential therapeutic applications.
Structural Features
Pratensein belongs to the class of 7-hydroxyisoflavones in which the isoflavone scaffold is substituted by hydroxyl groups at positions 5, 7, and 3′, with a methoxy group at position 4′ . This specific substitution pattern distinguishes pratensein from other related isoflavones and contributes to its unique biochemical interactions. It is classified as both a member of 7-hydroxyisoflavones and 4′-methoxyisoflavones, representing a conjugate acid of pratensein(1-) . The structural characteristics of pratensein enable its interaction with various biological targets, contributing to its diverse pharmacological activities.
Natural Sources and Distribution
Plant Sources
Pratensein has been isolated from various plant species, most notably from Trifolium pratense L. (red clover) of the Leguminosae family . This compound is an important constituent of red clover's isoflavone profile, which includes other bioactive isoflavones such as biochanin A, formononetin, daidzein, and genistein . Additionally, pratensein has been reported in other plant species including Cicer microphyllum and Dalbergia sissoo . It has also been identified in Cuscuta kotchiana, from which both pratensein and its glycoside form have been isolated for anticancer studies .
Extraction and Isolation
The isolation of pratensein from natural sources typically involves extraction with hydroalcoholic solvents followed by various chromatographic techniques. The original isolation and structural elucidation of pratensein from red clover was reported by E. Wong in 1963 . Alternative synthetic approaches have also been developed by researchers such as A.C. Jain and P.K. Bambab in 1987 . The compound may also exist as a glycoside, with pratensein 7-O-glucoside (C22H22O11, molecular weight 462.4 g/mol) being a notable derivative .
Biological Activities
Effects on Breast Cancer
Research has demonstrated significant anticancer activity of pratensein against breast cancer cell lines. In studies with MCF-7 (estrogen receptor-positive) and MDA-MB-231 cells, pratensein reduced cell viability in a dose- and time-dependent manner . The compound induces both apoptosis and autophagy in these cells, as evidenced by changes in expression levels of key regulatory genes. Specifically, pratensein treatment upregulates p53, BAX, and caspase-3 expression while downregulating BCL-2 expression . These molecular changes are consistent with the induction of programmed cell death pathways.
Additionally, pratensein has demonstrated synergistic effects when combined with tamoxifen, a standard therapeutic agent for estrogen receptor-positive breast cancer . This synergism suggests potential applications as an adjuvant in conventional breast cancer therapy, potentially allowing for dose reduction of chemotherapeutic agents and minimized side effects.
Effects on Prostate Cancer
Pratensein, as a component of Trifolium pratense extract, has shown promising effects against prostate cancer cell lines. Studies on LNCap and PC3 prostate cancer cells demonstrate significant reductions in cell viability after treatment with T. pratense extract containing pratensein . The extract induced apoptosis, as confirmed by Acridine Orange/Ethidium Bromide (AO/EB) staining. At the molecular level, the treatment led to:
-
Upregulation of p53, Bax, and caspase-3, 8, and 9 genes
-
Downregulation of anti-apoptotic Bcl-2 gene expression
-
Increased expression of autophagy-related genes LC3, beclin-1, and ATG-7
Furthermore, the extract significantly decreased nitric oxide (NO) production in a dose-dependent manner, which may contribute to its anticancer effects . When combined with paclitaxel, a standard chemotherapeutic agent, the extract containing pratensein demonstrated synergistic cytotoxic effects, suggesting potential benefits in combination therapy approaches.
Effects on Glioblastoma
Studies on glioblastoma multiforme (GBM) cells have shown that extracts containing pratensein can induce both apoptosis and autophagy cell death. In U87MG glioblastoma cells, treatment with T. pratense extract significantly reduced cell viability in a time- and dose-dependent manner. The extract also decreased nitric oxide production by these cells . At the molecular level, the treatment increased the expression of p53, BAX, caspase 3, LC3, beclin-1, and ATG-7, while reducing BCL-2 expression . These findings suggest potential applications for pratensein-containing extracts in the management of aggressive brain tumors.
When combined with temozolomide (TMZ), the standard chemotherapeutic agent for GBM, the extract demonstrated synergistic cytotoxic effects, suggesting potential benefits in combination therapy approaches .
Protection Against Ischemia-Reperfusion Injury
Pratensein (PTS) has demonstrated significant cardioprotective effects in models of myocardial ischemia-reperfusion (I/R) injury. In H9c2 cardiomyocyte cells subjected to oxygen-glucose deprivation/reoxygenation (OGD/R), pratensein treatment reduced apoptosis and improved cell viability . The compound attenuated the increase in reactive oxygen species (ROS) and malondialdehyde (MDA) production typically associated with OGD/R injury. Additionally, pratensein prevented the decrease in glutathione (GSH) content caused by OGD/R, further supporting its antioxidant properties .
Antioxidant and Anti-ferroptotic Mechanisms
One of the key mechanisms underlying pratensein's cardioprotective effects is its modulation of the Nrf2/GPX4 signaling pathway. The study by researchers revealed that the decreased expression levels of Nrf2 and GPX4 in OGD/R-treated H9c2 cells were significantly elevated after pratensein treatment . The compound also restrained the increased Fe2+ levels in OGD/R-treated cells, suggesting anti-ferroptotic activity.
Knockdown of Nrf2 in H9c2 cells reversed the protective effect of pratensein on ferroptosis induced by OGD/R, indicating that this pathway is essential for the compound's cardioprotective action. These findings suggest that pratensein may protect cardiomyocytes from OGD/R-caused ferroptosis by specifically modulating the Nrf2/GPX4 signaling pathway .
Cholesterol Regulation
Pratensein has demonstrated potential benefits for cholesterol metabolism through its effects on scavenger receptor class B type I (SR-BI) and its human homologue CLA-1, which play important roles in reverse cholesterol transport (RCT) as high-density lipoprotein (HDL) receptors.
In a cell-based screening model, pratensein was shown to upregulate CLA-1 transcriptional activity . This effect was demonstrated at both mRNA and protein levels and validated by increased uptake of 1,1'-dioctadecyl-3,3,3',3'-tetramethylindocarbocyanine perchlorate-labeled (DiI)-HDL in HepG2 cells . The cis-elements responsible for pratensein's upregulatory effects were mapped to the -1055/-182 bp fragment of the CLA-1 promoter in HepG2 cells.
These findings provide a potential molecular mechanism by which pratensein and other isoflavones might prevent atherosclerosis, suggesting applications in cardiovascular health beyond direct cardioprotection .
Neurological Effects
Pratensein has shown promising effects on cognitive function. Studies indicate that this flavonoid can improve β-amyloid-induced cognitive impairment in rats by reducing oxidative damage and restoring synapses and brain-derived neurotrophic factor (BDNF) levels . These findings suggest potential applications for pratensein in neurodegenerative disorders characterized by oxidative stress and synaptic dysfunction.
Structure-Activity Relationships
The biological activities of pratensein can be partially attributed to its specific structural features. As an isoflavone with a particular hydroxylation and methoxylation pattern, it shares structural similarities with other bioactive isoflavones such as biochanin A, formononetin, genistein, and daidzein, which have also demonstrated various biological activities .
The presence of hydroxyl groups at positions 5, 7, and 3' contributes to its antioxidant properties, while the methoxy group at position 4' may influence its binding to specific biological targets. Structure-activity relationship studies comparing pratensein with other related isoflavones could provide valuable insights into the specific structural determinants of its various biological activities.
These synergistic effects suggest potential applications for pratensein as an adjuvant in cancer therapy, potentially allowing for dose reduction of conventional chemotherapeutic agents and minimized side effects. The combination index (CI) and dose reduction index (DRI) values calculated in these studies support the potential clinical utility of such combination approaches .
Future Research Directions
Given the diverse biological activities of pratensein, several promising research directions emerge:
-
Further elucidation of molecular mechanisms underlying its anticancer activities
-
Development of semi-synthetic derivatives with enhanced potency or bioavailability
-
Clinical evaluation of pratensein-containing formulations as adjuvants in cancer therapy
-
Investigation of potential applications in neurodegenerative disorders
-
Exploration of its cardioprotective effects in in vivo models of myocardial infarction
Mass Molarity Calculator
- mass of a compound required to prepare a solution of known volume and concentration
- volume of solution required to dissolve a compound of known mass to a desired concentration
- concentration of a solution resulting from a known mass of compound in a specific volume