Esermethole
CAS No.: 65166-97-4
Cat. No.: VC21347575
Molecular Formula: C14H20N2O
Molecular Weight: 232.32 g/mol
* For research use only. Not for human or veterinary use.
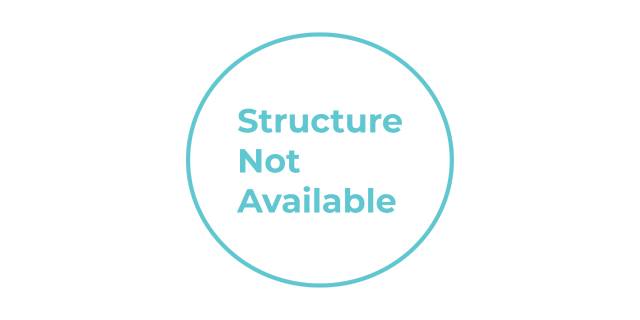
Specification
CAS No. | 65166-97-4 |
---|---|
Molecular Formula | C14H20N2O |
Molecular Weight | 232.32 g/mol |
IUPAC Name | (3aR,8bS)-7-methoxy-3,4,8b-trimethyl-2,3a-dihydro-1H-pyrrolo[2,3-b]indole |
Standard InChI | InChI=1S/C14H20N2O/c1-14-7-8-15(2)13(14)16(3)12-6-5-10(17-4)9-11(12)14/h5-6,9,13H,7-8H2,1-4H3/t13-,14+/m1/s1 |
Standard InChI Key | WKJDLYATGMSVOQ-KGLIPLIRSA-N |
Isomeric SMILES | C[C@@]12CCN([C@@H]1N(C3=C2C=C(C=C3)OC)C)C |
SMILES | CC12CCN(C1N(C3=C2C=C(C=C3)OC)C)C |
Canonical SMILES | CC12CCN(C1N(C3=C2C=C(C=C3)OC)C)C |
Introduction
Property | Value |
---|---|
IUPAC Name | (3aR,8bS)-7-methoxy-3,4,8b-trimethyl-2,3a-dihydro-1H-pyrrolo[2,3-b]indole |
Molecular Formula | C₁₄H₂₀N₂O |
Molecular Weight | 232.32 g/mol |
CAS Number | 65166-97-4 |
Appearance | Not explicitly stated in literature |
Stereochemistry | Contains (3aR,8bS) stereochemistry |
InChI | InChI=1S/C14H20N2O/c1-14-7-8-15(2)13(14)16(3)12-6-5-10(17-4)9-11(12)14/h5-6,9,13H,7-8H2,1-4H3/t13-,14+/m1/s1 |
Canonical SMILES | CC12CCN(C1N(C3=C2C=C(C=C3)OC)C)C |
The compound features a tricyclic structure with two nitrogen atoms in its core scaffold. The methoxy group at position 7 provides distinctive chemical reactivity, while the three methyl groups contribute to its lipophilicity and conformational properties .
Structural Characteristics
Esermethole's structure consists of a hexahydropyrrolo[2,3-b]indole skeleton with specific substituents that define its chemical identity. X-ray crystallographic studies have provided detailed insights into the three-dimensional arrangement of atoms within the molecule . The compound possesses a complex tricyclic framework with a characteristic pyrroloindoline core that contains two adjacent nitrogen atoms, one of which is part of an indole moiety.
The conformational flexibility of esermethole is relatively constrained due to its rigid tricyclic framework. This structural rigidity is advantageous for specific molecular recognition events in biological systems, potentially explaining its diverse biological activities.
Synthesis Methods
The synthesis of esermethole has been approached through various strategies, reflecting its importance as both a target molecule and a synthetic intermediate. Three major approaches have emerged in the literature: asymmetric synthesis methods, palladium-catalyzed reactions, and cycloaddition-based strategies.
Asymmetric Synthesis
A significant asymmetric synthesis of esermethole was reported by Pallavicini et al., featuring the asymmetric alkylation at C(3) of racemic 1,3-dimethyl-5-methoxyoxindole . This approach employed chiral alkylating agents derived from (-)-menthol and (S)-N-methyl-(1-phenylethyl) amine. The reaction of the latter with the oxindole substrate in toluene at 10°C, in the presence of t-butyllithium, yielded (3S,1'S)-N-methyl-N-(1'-phenylethyl)-1,3-dimethyl-5-methoxyoxindol-3-ilacetamide with a 63% diastereomeric excess. The desired diastereomer was separated from the minor (3R,1'S) counterpart and subsequently converted to (-)-esermethole with 99.6% enantiomeric excess in two synthetic steps .
This approach is particularly valuable as it establishes the critical stereocenter at C3, which ultimately controls the stereochemistry of the pyrroloindoline core in the final product. The use of chiral auxiliaries enabled the stereoselective formation of this quaternary center, providing access to enantiomerically pure esermethole.
Palladium-Catalyzed Methods
Zhou et al. developed an efficient synthetic route employing palladium-catalyzed sequential arylation-allylation of o-bromoanilides . This methodology enables the construction of oxindoles bearing a fully substituted carbon quaternary center at the C3 position, which serves as a key intermediate in esermethole synthesis.
Remarkably, this method utilizes inexpensive triphenylphosphine as an effective ligand, making it particularly attractive for practical applications. The approach allows for the preparation of various esermethole analogues by modifying the starting materials, demonstrating its versatility for accessing structurally related compounds .
Another palladium-catalyzed approach was reported by Wang et al., utilizing phosphine-catalyzed allylic alkylation of (hetero)aryl alkynes with pronucleophiles . This atom-economical method provided a concise route to (±)-esermethole with broad substrate compatibility and potential for scale-up.
Cycloaddition Reactions
Ozawa et al. reported a synthesis of (±)-esermethole using an intramolecular carbamoylketene-alkene [2+2] cycloaddition followed by nitrone-mediated regioselective ring expansion . This approach provides an elegant strategy for constructing the basic carbon framework of esermethole.
The key step involves the generation of a carbamoylketene intermediate that undergoes an intramolecular [2+2] cycloaddition with a tethered alkene. The resulting cyclobutanone intermediate then undergoes a nitrone-mediated ring expansion to establish the pyrroloindoline core structure. This methodology offers a unique approach to assembling the complex heterocyclic architecture of esermethole through the strategic use of cycloaddition chemistry .
Another innovative approach involves a palladium-catalyzed enantioselective domino Heck-cyanation sequence, as described by Lu and colleagues . This method utilizes nontoxic potassium ferrocyanide as a cyanide source and employs (S)-DIFLUORPHOS as a chiral supporting ligand, achieving enantioselectivities up to 79% ee. The resulting 3-alkyl-3-cyanomethyl-2-oxindole intermediates are then transformed into esermethole through a series of functional group manipulations. This approach represents a concise total synthesis of both esermethole and physostigmine .
Table 2: Comparison of Key Synthetic Methods for Esermethole
Biological Activity
Esermethole exhibits several significant biological activities that contribute to its potential therapeutic applications. Research has demonstrated its effects in various biological systems, including neuroprotection, antitumor activity, and antimicrobial properties.
Neuroprotective Effects
Esermethole has demonstrated promising neuroprotective properties, particularly in models of oxidative stress-induced neuronal damage. Studies suggest that the compound reduces apoptosis and oxidative stress in neuronal cells, potentially offering benefits for neurodegenerative conditions. Its structural relationship to physostigmine, which is known for acetylcholinesterase inhibition, suggests that esermethole may share some mechanistic features related to cholinergic transmission modulation.
The neuroprotective effects of esermethole are particularly significant in the context of neurodegenerative diseases such as Alzheimer's disease, where cholinergic deficits play a prominent role in pathogenesis. By enhancing cholinergic transmission through acetylcholinesterase inhibition, compounds like esermethole and its derivative physostigmine may help alleviate cognitive symptoms associated with these conditions .
Antimicrobial Properties
Esermethole has demonstrated activity against certain bacterial strains, particularly Gram-positive bacteria. This antimicrobial activity expands the potential therapeutic applications of esermethole beyond neurological and oncological contexts. The compound's ability to inhibit bacterial growth suggests potential utility in addressing infectious diseases, particularly in an era of increasing antimicrobial resistance.
Table 3: Biological Activities of Esermethole
Biological Activity | Effect | Potential Applications |
---|---|---|
Neuroprotection | Reduces apoptosis and oxidative stress in neuronal cells | Neurodegenerative diseases (e.g., Alzheimer's disease) |
Antitumor | Inhibits growth in HeLa cell lines | Cancer therapy, particularly for cervical cancer |
Antimicrobial | Activity against Gram-positive bacteria | Infectious disease treatment |
Relationship to Physostigmine
Esermethole serves as a crucial synthetic precursor to physostigmine, a well-established acetylcholinesterase inhibitor with significant clinical applications . Physostigmine, isolated from the Calabar bean (Physostigma venenosum), is an important anticholinesterase compound with several clinical uses and is considered a promising therapeutic agent for Alzheimer's disease .
The conversion of esermethole to physostigmine typically involves a two-step sequence, which has been well-documented in the literature . This transformation introduces the carbamate functionality characteristic of physostigmine while preserving the critical stereochemical features established in the esermethole scaffold.
Physostigmine exerts its pharmacological effects primarily through inhibition of acetylcholinesterase, the enzyme responsible for hydrolyzing the neurotransmitter acetylcholine in the synaptic cleft. By inhibiting this enzyme, physostigmine increases acetylcholine levels and enhances cholinergic neurotransmission, which is particularly beneficial in conditions characterized by cholinergic deficits, such as Alzheimer's disease .
The structural relationship between esermethole and physostigmine highlights the importance of the pyrroloindoline core as a pharmacophore for acetylcholinesterase inhibition. The specific stereochemistry and substitution pattern established in esermethole provide the foundation for physostigmine's biological activity, emphasizing the significance of stereoselective synthetic approaches to esermethole production.
Applications and Future Directions
Esermethole possesses several potential applications across multiple fields, from synthetic organic chemistry to medicinal chemistry and drug development. Its primary importance lies in its role as a key intermediate in the synthesis of physostigmine and related alkaloids with therapeutic potential .
In synthetic chemistry, esermethole serves as a valuable model system for developing and refining stereoselective synthetic methodologies, particularly those involving the construction of quaternary stereocenters. The diverse synthetic approaches documented in the literature reflect ongoing efforts to optimize the production of esermethole and related compounds through more efficient, sustainable, and stereoselective methods .
From a medicinal chemistry perspective, esermethole's diverse biological activities position it as a promising lead compound for drug discovery efforts. Its neuroprotective, antitumor, and antimicrobial properties provide multiple avenues for therapeutic development. Structure-activity relationship studies based on the esermethole scaffold could yield novel derivatives with enhanced potency, selectivity, and pharmacokinetic properties.
Future research directions may include:
-
Development of more efficient and highly enantioselective synthetic routes to esermethole, potentially employing emerging catalytic methods and sustainable chemistry principles.
-
Comprehensive evaluation of esermethole's mechanism of action across various biological systems, with particular emphasis on its neuroprotective and antitumor effects.
-
Design and synthesis of esermethole analogues with optimized properties for specific therapeutic applications, such as enhanced blood-brain barrier penetration for neurological indications.
-
Exploration of esermethole's potential in combination therapies, particularly for complex conditions like neurodegenerative diseases and cancer.
- mass of a compound required to prepare a solution of known volume and concentration
- volume of solution required to dissolve a compound of known mass to a desired concentration
- concentration of a solution resulting from a known mass of compound in a specific volume