Finasteride Carboxylic Acid
CAS No.: 116285-37-1
Cat. No.: VC21352618
Molecular Formula: C23H34N2O4
Molecular Weight: 402.5 g/mol
Purity: > 95%
* For research use only. Not for human or veterinary use.
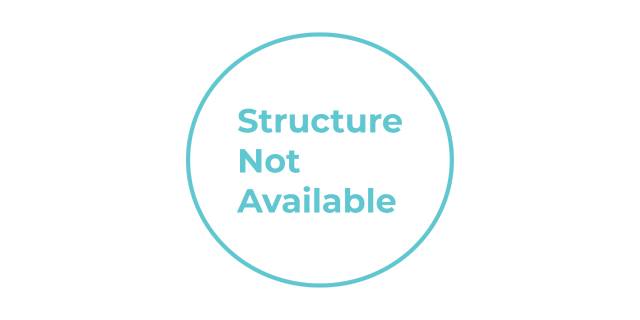
Specification
CAS No. | 116285-37-1 |
---|---|
Molecular Formula | C23H34N2O4 |
Molecular Weight | 402.5 g/mol |
IUPAC Name | 2-[[(1S,3aS,3bS,5aR,9aR,9bS,11aS)-9a,11a-dimethyl-7-oxo-1,2,3,3a,3b,4,5,5a,6,9b,10,11-dodecahydroindeno[5,4-f]quinoline-1-carbonyl]amino]-2-methylpropanoic acid |
Standard InChI | InChI=1S/C23H34N2O4/c1-21(2,20(28)29)25-19(27)16-7-6-14-13-5-8-17-23(4,12-10-18(26)24-17)15(13)9-11-22(14,16)3/h10,12-17H,5-9,11H2,1-4H3,(H,24,26)(H,25,27)(H,28,29)/t13-,14-,15-,16+,17+,22-,23+/m0/s1 |
Standard InChI Key | OFTBMAPJHKDDJV-MKMSXTRJSA-N |
Isomeric SMILES | C[C@]12CC[C@H]3[C@H]([C@@H]1CC[C@@H]2C(=O)NC(C)(C)C(=O)O)CC[C@@H]4[C@@]3(C=CC(=O)N4)C |
SMILES | CC12CCC3C(C1CCC2C(=O)NC(C)(C)C(=O)O)CCC4C3(C=CC(=O)N4)C |
Canonical SMILES | CC12CCC3C(C1CCC2C(=O)NC(C)(C)C(=O)O)CCC4C3(C=CC(=O)N4)C |
Appearance | Off-White to Pale Yellow Solid |
Introduction
Mechanism of Action
Inhibition of 5α-Reductase
Finasteride Carboxylic Acid functions as a potent inhibitor of the enzyme 5-alpha reductase . This enzyme plays a crucial role in androgen metabolism, being responsible for the conversion of testosterone to dihydrotestosterone (DHT) . By inhibiting this enzymatic activity, Finasteride Carboxylic Acid effectively reduces the levels of DHT in the body, which has significant implications for conditions associated with elevated DHT levels . The inhibition occurs at the molecular level, with Finasteride Carboxylic Acid binding to the active site of 5-alpha reductase, thereby preventing the conversion of testosterone to DHT .
The mechanism of action of Finasteride Carboxylic Acid is particularly relevant in the context of understanding hormonal regulation and intervention. The specificity of its binding to the 5-alpha reductase enzyme represents a targeted approach to modulating androgen activity, which has been leveraged in therapeutic applications . The effectiveness of this inhibition is determined by various factors, including the binding affinity of the compound to the enzyme and the concentration of the compound at the site of action.
Comparison with Parent Compound
When compared to its parent compound finasteride, Finasteride Carboxylic Acid exhibits certain differences in its pharmacological profile while maintaining the core mechanism of 5-alpha reductase inhibition. Studies have shown that finasteride itself was initially developed based on understanding the physiological role of testosterone in prostate growth and the identification of steroid 5α-reductase type II deficiency . The development of finasteride as a chemopreventive agent for prostate cancer has been well documented, with significant clinical trials demonstrating its efficacy in reducing prostate cancer risk .
Metabolism and Pharmacokinetics
Metabolic Pathway Formation
The biotransformation of finasteride to Finasteride Carboxylic Acid involves a complex series of enzymatic reactions categorized into Phase I and Phase II metabolism . In Phase I metabolism, finasteride undergoes hydroxylation and oxidation reactions mediated by cytochrome P450 enzymes, particularly CYP3A4 . This process initially converts finasteride to ω-hydroxyfinasteride through hydroxylation of the t-butyril group . Following this, further oxidation occurs, leading to the formation of finasteride-ω-oic acid, which is essentially Finasteride Carboxylic Acid .
Phase II metabolism involves glucuronidation, a conjugation pathway that significantly enhances the water solubility of the compound . This process is catalyzed by UDP-glucuronosyltransferase (UGT) enzymes, with UGT1A3 being specifically identified as responsible for the glucuronidation of finasteride-ω-oic acid . The addition of polar glucuronic acid to the substrate facilitates excretion via urine or bile, representing an important mechanism for eliminating the compound from the body . The effectiveness of this metabolic pathway can vary between individuals due to genetic factors affecting enzyme expression and activity.
Genetic Variations Affecting Metabolism
Genetic variations in the enzymes involved in finasteride metabolism can significantly impact the formation and elimination of Finasteride Carboxylic Acid. The CYP3A subfamily, which includes CYP3A4, CYP3A5, CYP3A7, and CYP3A43, exhibits high sequence homology (84%) and shows overlap in substrate specificity, particularly between CYP3A4 and CYP3A5 . Interindividual variation in CYP3A enzyme activity can be as high as tenfold in vivo, with genetic variability accounting for up to 90% of this observed variation .
Studies have identified specific single nucleotide polymorphisms (SNPs) in the CYP3A4 gene that can either increase or decrease the 6β-hydroxylation of testosterone, which may parallel changes in finasteride metabolism . Similarly, variations in the UGT enzymes responsible for glucuronidation can affect the conversion of finasteride metabolites, potentially altering the therapeutic efficacy and side effect profile of the drug . Understanding these genetic factors is crucial for predicting individual responses to finasteride treatment and optimizing dosing strategies to maximize benefits while minimizing adverse effects.
Synthesis Methods
Industrial Synthesis Approach
The synthesis of finasteride, which ultimately leads to the formation of Finasteride Carboxylic Acid through metabolism, involves several chemical transformations starting from readily available precursors. One documented method utilizes progesterone as the raw material for synthesizing 3-carbonyl-4-androstene-17β-carboxylic acid in a single step through a bromoform reaction . This approach involves dissolving progesterone in dioxane and adding bromine (Br2) and 15% sodium hydroxide aqueous solution at a temperature of 0-5°C, followed by a 6-hour reaction period . After completion, the reaction mixture is poured into ice water, then extracted, washed, dried, and concentrated under reduced pressure to yield white crystals of 3-carbonyl-4-androstene-17β-carboxylic acid .
The industrial synthesis process continues through several additional steps to produce finasteride . This improved synthesis method achieves a total yield of 24.2% and offers several advantages for commercial production . The process utilizes relatively inexpensive starting materials, provides stable yields, and is suitable for industrial-scale production, with the final product meeting pharmacopeial quality standards . The synthesis method also incorporates environmentally friendly modifications, such as replacing more toxic reagents like oxalyl chloride with thionyl chloride, and substituting benzene selenous anhydride with 2,3-dichloro-5,6-dicyano-p-benzoquinone (DDQ) and bis(trimethylsilyl)trifluoroacetamide (BSTFA) .
Clinical Significance and Applications
Biochemical Research Applications
Beyond its therapeutic applications, Finasteride Carboxylic Acid serves as an important tool in biochemical research focused on understanding androgen metabolism and regulation. As a metabolite with defined structural characteristics and inhibitory properties, it provides insights into the biochemical pathways involved in the regulation of androgenic hormones . Studying the mechanism of action of Finasteride Carboxylic Acid can enhance our understanding of these pathways and potentially lead to the development of more selective and effective therapeutic agents .
Research into the pharmacogenetics of finasteride metabolism, including the formation and activity of Finasteride Carboxylic Acid, represents an emerging area of investigation with implications for personalized medicine . By identifying genetic factors that influence drug metabolism and response, researchers aim to develop tailored treatment approaches that maximize efficacy while minimizing adverse effects . The discovery of novel finasteride metabolites, including Phase I and Phase II products, suggests potential alternative pathways of metabolism that warrant further exploration . These investigations may lead to the identification of new biomarkers for treatment response or reveal additional therapeutic targets for intervention.
Data Table: Properties and Characteristics of Finasteride Carboxylic Acid
- mass of a compound required to prepare a solution of known volume and concentration
- volume of solution required to dissolve a compound of known mass to a desired concentration
- concentration of a solution resulting from a known mass of compound in a specific volume