1beta-Hydroxydeoxycholic acid
CAS No.: 80434-32-8
Cat. No.: VC21355008
Molecular Formula: C24H36D4O5
Molecular Weight: 408.6 g/mol
Purity: 95% by NMR; 98% atom D
* For research use only. Not for human or veterinary use.
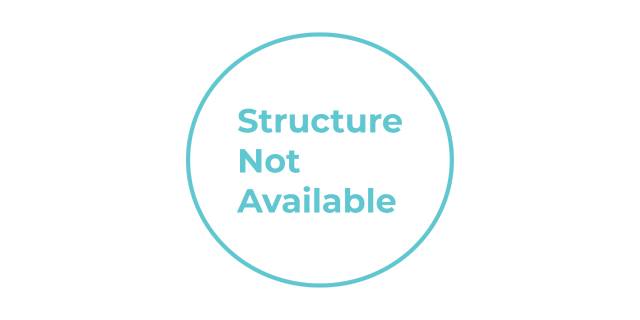
Specification
CAS No. | 80434-32-8 |
---|---|
Molecular Formula | C24H36D4O5 |
Molecular Weight | 408.6 g/mol |
IUPAC Name | (4R)-4-[(1R,3S,5R,8S,9S,10S,12S,13R,14S,17R)-1,3,12-trihydroxy-10,13-dimethyl-2,3,4,5,6,7,8,9,11,12,14,15,16,17-tetradecahydro-1H-cyclopenta[a]phenanthren-17-yl]pentanoic acid |
Standard InChI | InChI=1S/C24H40O5/c1-13(4-9-22(28)29)17-7-8-18-16-6-5-14-10-15(25)11-20(26)23(14,2)19(16)12-21(27)24(17,18)3/h13-21,25-27H,4-12H2,1-3H3,(H,28,29)/t13-,14-,15+,16+,17-,18+,19+,20-,21+,23+,24-/m1/s1 |
Standard InChI Key | DAKYVYUAVGJDRK-FPUZENINSA-N |
Isomeric SMILES | C[C@H](CCC(=O)O)[C@H]1CC[C@@H]2[C@@]1([C@H](C[C@H]3[C@H]2CC[C@H]4[C@@]3([C@@H](C[C@H](C4)O)O)C)O)C |
SMILES | CC(CCC(=O)O)C1CCC2C1(C(CC3C2CCC4C3(C(CC(C4)O)O)C)O)C |
Canonical SMILES | CC(CCC(=O)O)C1CCC2C1(C(CC3C2CCC4C3(C(CC(C4)O)O)C)O)C |
Introduction
Chemical Structure and Properties
Molecular Characteristics
1beta-Hydroxydeoxycholic acid is a trihydroxylated bile acid derivative with a molecular formula of C24H40O5 and a molecular weight of approximately 408.6 g/mol. Its structure features hydroxyl groups at the 1β, 3α, and 12α positions of the cholanic acid backbone. The compound exists primarily as 1beta-hydroxydeoxycholate (C24H39O5-) at physiological pH, which is the conjugate base formed by deprotonation of the carboxyl group . This structure contributes to its physiochemical properties and biological functions.
Related Compounds and Derivatives
The compound belongs to the family of cholanic acids and is closely related to deoxycholic acid (DCA), from which it is derived through 1β-hydroxylation. It has several chemical designations including 1beta,3alpha,12alpha-trihydroxy-5beta-cholan-24-oate in its ionized form . In biological systems, 1β-OH-DCA often exists as conjugates with amino acids such as glycine and taurine, forming glyco-1β-OH-DCA and tauro-1β-OH-DCA, respectively. These conjugates play important roles in the compound's excretion and distribution within the body.
Metabolism and Biosynthesis
CYP3A-Mediated Formation
The formation of 1β-OH-DCA occurs primarily through the hydroxylation of deoxycholic acid (DCA) at the 1β position, a reaction that is selectively mediated by CYP3A enzymes . This metabolic pathway is significant because the CYP3A family, particularly CYP3A4, is responsible for the metabolism of approximately 50% of clinically used drugs. The selectivity of this reaction makes 1β-OH-DCA particularly valuable as a biomarker for CYP3A activity. Research has demonstrated that the metabolic conversion of DCA to 1β-OH-DCA is sufficiently sensitive to reflect changes in CYP3A activity resulting from enzyme inhibition or induction .
Excretion and Distribution
After formation, 1β-OH-DCA undergoes conjugation with glycine or taurine before excretion. These conjugates are found in both plasma and urine, with studies detecting them in quantifiable amounts in both matrices . The compound and its conjugates are excreted primarily via urine, making spot urine collection a viable non-invasive sampling method for monitoring CYP3A activity . The half-life of urinary endogenous 1β-OH-DCA/total DCA ratio (1β-OH-DCA/ToDCA) has been estimated at approximately four days, which is considerably shorter than some other endogenous CYP3A biomarkers .
Analytical Methods for Detection
Chromatographic Techniques
Multiple analytical methods have been developed for the detection and quantification of 1β-OH-DCA in biological samples. For chromatographic separation, studies have utilized various approaches including:
-
Phenomenex Synergi™ 4 μm Polar-RP 80 Å LC Column (150 × 2 mm) with a mobile phase consisting of 10 mM ammonium formate in 5% methanol and acetonitrile
-
Acquity UPLC BEH C18 column (2.1 × 100 mm, 1.7 μm) with a mobile phase of 0.1% formic acid aqueous solution and acetonitrile
These methods allow effective separation of 1β-OH-DCA from related compounds and matrix components, which is crucial for accurate quantification.
Mass Spectrometry Methods
Mass spectrometry has been the detection method of choice for 1β-OH-DCA analysis due to its high sensitivity and selectivity. Research has employed various approaches including:
-
Liquid chromatography-tandem mass spectrometry (LC-MS/MS) with turbo ion spray interphase operated in positive ion mode
-
Ultra-performance liquid chromatography-high resolution mass spectrometry (UPLC-HRMS) using a Synapt G2-Si Q-TOF mass spectrometer
-
LC/tandem mass spectrometry for the identification of glyco- and tauro-conjugates of the compound
A particularly sensitive LC-MS/MS method with a lower limit of quantitation of 50 pg/ml has been developed, enabling the measurement of basal levels of 1β-OH-DCA and its conjugates in plasma, as well as detecting reductions in these levels following CYP3A inhibition .
Clinical Applications as a Biomarker
Assessment of CYP3A Inhibition
One of the most promising applications of 1β-OH-DCA is in the assessment of CYP3A inhibition in drug-drug interaction studies. Research has demonstrated that the 1β-OH-DCA/ToDCA ratio in urine responds sensitively to CYP3A inhibitors of varying potency . Table 1 summarizes the effects of different CYP3A inhibitors on 1β-OH-DCA levels.
Table 1: Effects of CYP3A Inhibitors on 1β-OH-DCA Levels
The data indicates that 1β-OH-DCA responds to CYP3A inhibition in a manner consistent with the known potency of these inhibitors, supporting its utility as a biomarker for CYP3A activity.
Assessment of CYP3A Induction
In addition to inhibition, 1β-OH-DCA also responds to CYP3A induction. Studies have shown that rifampin, a potent CYP3A inducer, leads to substantial increases in 1β-OH-DCA and its conjugates in plasma . Specifically, rifampin induction resulted in:
-
6.8-fold increase in area under the curve from time of dosing to the last measurable concentration (AUC LST)
-
7.8-fold increase in area under the curve from time of dosing to 24 hours (AUC 24h)
-
8.3-fold increase in maximum concentration (Cmax)
-
10.3-fold increase in mean concentrations for total 1β-OH-DCA
These findings indicate that 1β-OH-DCA can effectively capture both inhibition and induction of CYP3A activity, making it a versatile biomarker for comprehensive DDI assessment.
Comparison with Other Biomarkers
1β-OH-DCA offers several advantages over other endogenous biomarkers of CYP3A activity, particularly 4β-hydroxycholesterol (4β-HC). Table 2 compares key characteristics of these biomarkers.
Table 2: Comparison of 1β-OH-DCA and 4β-HC as CYP3A Biomarkers
Characteristic | 1β-OH-DCA | 4β-HC | Advantage |
---|---|---|---|
Half-life | ~4 days | Long (17-21 days) | 1β-OH-DCA (shorter half-life allows faster response) |
Sensitivity to inhibition | High | Limited | 1β-OH-DCA (can detect CYP3A inhibition) |
Sensitivity to induction | High | Moderate | 1β-OH-DCA (larger dynamic range) |
Matrices | Plasma and urine | Primarily plasma | 1β-OH-DCA (multiple sampling options) |
Non-invasiveness | High (when using urine) | Moderate | 1β-OH-DCA (urine collection is non-invasive) |
The key advantage of 1β-OH-DCA over 4β-HC is its ability to detect CYP3A inhibition, which is limited with 4β-HC due to its long half-life and narrow dynamic range . Additionally, the shorter half-life of 1β-OH-DCA allows for more rapid assessment of changes in CYP3A activity.
Developmental and Age-Related Aspects
Presence in Infants
Interestingly, 1β-hydroxylated bile acids, including 1β-hydroxyl-cholic acid (CA-1β-ol) and 1β-hydroxyl-chenodeoxycholic acid (CDCA-1β-ol), have been detected in relatively large amounts in the urine of infants . These 1β-hydroxylated bile acids are conjugated with amino acids, particularly taurine, before excretion via urine. They represent predominant bile acids during infancy, suggesting developmental differences in bile acid metabolism .
Differences Between Infant and Adult Metabolism
Research has indicated that the formation rates of 1β-hydroxylated bile acids in infant hepatocytes are higher than those in adult hepatocytes . This suggests developmental regulation of the enzymes involved in 1β-hydroxylation of bile acids. The specific mechanisms underlying these differences remain unclear, but they highlight the importance of considering age-related factors when using 1β-OH-DCA as a biomarker of CYP3A activity.
- mass of a compound required to prepare a solution of known volume and concentration
- volume of solution required to dissolve a compound of known mass to a desired concentration
- concentration of a solution resulting from a known mass of compound in a specific volume