SN 2
CAS No.: 823218-99-1
Cat. No.: VC21409466
Molecular Formula: C17H21NO
Molecular Weight: 255.35 g/mol
* For research use only. Not for human or veterinary use.
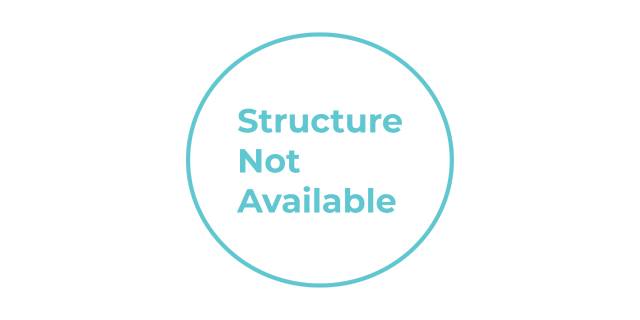
Specification
CAS No. | 823218-99-1 |
---|---|
Molecular Formula | C17H21NO |
Molecular Weight | 255.35 g/mol |
IUPAC Name | 5-(2,4,6-trimethylphenyl)-3-oxa-4-azatricyclo[5.2.1.02,6]dec-4-ene |
Standard InChI | InChI=1S/C17H21NO/c1-9-6-10(2)14(11(3)7-9)16-15-12-4-5-13(8-12)17(15)19-18-16/h6-7,12-13,15,17H,4-5,8H2,1-3H3 |
Standard InChI Key | WKLZNTYMDOPBSE-UHFFFAOYSA-N |
SMILES | CC1=CC(=C(C(=C1)C)C2=NOC3C2C4CCC3C4)C |
Canonical SMILES | CC1=CC(=C(C(=C1)C)C2=NOC3C2C4CCC3C4)C |
Introduction
Fundamentals of SN2 Reactions
Definition and Nomenclature
The term "SN2" refers to a specific type of reaction mechanism where "S" stands for substitution, "N" for nucleophilic, and "2" indicates the bimolecular nature of the reaction. In the Hughes-Ingold classification system, this designation signifies that both the nucleophile and substrate are involved in the rate-determining step. This distinguishes SN2 from the SN1 mechanism, where the displacement of the leaving group occurs in a separate step before nucleophilic attack .
Mechanistic Details
The SN2 reaction occurs predominantly at an aliphatic sp³-hybridized carbon center bearing an electronegative, stable leaving group. The reaction proceeds through a concerted mechanism where the nucleophile forms a new bond with the carbon while the leaving group simultaneously detaches. This synchronous bond-making and bond-breaking process results in a distinct transition state where the central carbon is pentacoordinate and approximately sp²-hybridized .
A crucial characteristic of the SN2 mechanism is the backside attack by the nucleophile. For optimal orbital overlap, the nucleophile approaches the reaction center at 180° relative to the leaving group. This geometric requirement can be understood through molecular orbital theory as a HOMO-LUMO interaction between the nucleophile and substrate, where the nucleophile's occupied lone pair orbital donates electrons to the unfilled σ* antibonding orbital between the central carbon and the leaving group .
Stereochemical Consequences
The backside attack requirement of SN2 reactions leads to a predictable stereochemical outcome. When the reaction occurs at a stereocenter, a complete inversion of configuration takes place, often referred to as "Walden inversion." This stereochemical result provides a useful diagnostic tool for identifying SN2 mechanisms in laboratory settings .
Factors Influencing SN2 Reaction Rates
The efficiency of SN2 reactions is governed by several key factors, which are discussed below in order of decreasing importance.
Substrate Structure
The structure of the alkyl halide (substrate) plays the most critical role in determining SN2 reaction rates. The transition state in an SN2 reaction is highly crowded, with five groups surrounding the central carbon atom. This crowding leads to significant steric considerations .
The relative reactivity of different substrate types follows the order:
Methyl > Primary > Secondary >> Tertiary
In methyl substrates, with three hydrogen atoms surrounding the reaction center, steric hindrance is minimal, allowing for efficient nucleophilic attack. As the number of alkyl substituents increases, the steric congestion around the reaction center increases dramatically, inhibiting nucleophilic approach .
Table 1: Relative SN2 Reaction Rates Based on Substrate Type
Substrate Type | Relative Rate | Steric Environment | Suitability for SN2 |
---|---|---|---|
Methyl (CH₃X) | 30 | Minimal hindrance | Excellent |
Primary (RCH₂X) | 1 | Low hindrance | Very good |
Secondary (R₂CHX) | 0.03 | Moderate hindrance | Limited |
Tertiary (R₃CX) | ~0 | Severe hindrance | Not viable |
Nucleophile Strength
The intrinsic nucleophilicity of the attacking species significantly impacts SN2 reaction rates. Stronger nucleophiles, characterized by higher electron density and greater ability to donate electrons, lead to faster reactions. Nucleophilicity is influenced by several factors:
-
Basicity: Generally, stronger bases are better nucleophiles within the same row of the periodic table
-
Polarizability: More polarizable atoms (larger atoms) tend to be better nucleophiles
-
Charge: Negatively charged species are typically more nucleophilic than their neutral counterparts
-
Solvation: Less solvated nucleophiles demonstrate enhanced nucleophilicity
Leaving Group Stability
The ease with which the leaving group departs affects the activation energy of the SN2 reaction. Good leaving groups are generally those that form stable species upon departure, typically weak bases that can effectively stabilize the negative charge. Common leaving groups in order of increasing effectiveness include:
F⁻ < OH⁻ < OC(O)R < Cl⁻ < Br⁻ < I⁻ < TsO⁻ (tosylate)
Solvent Effects
The solvent environment can dramatically influence SN2 reaction rates through various mechanisms:
-
Protic solvents tend to solvate nucleophiles through hydrogen bonding, reducing their nucleophilicity
-
Polar aprotic solvents (DMSO, DMF, acetonitrile) preferentially solvate cations while leaving anions relatively unencumbered, enhancing their nucleophilicity
-
Solvation of the transition state can either stabilize or destabilize it relative to the reactants
Kinetic and Thermodynamic Aspects
Rate Law and Kinetics
The SN2 reaction follows second-order kinetics, with the rate dependent on the concentrations of both the nucleophile and the substrate:
Rate = k[Nucleophile][Substrate]
This rate equation directly reflects the bimolecular nature of the reaction mechanism, where both species must collide for the reaction to proceed. The second-order kinetics serve as a crucial experimental criterion for identifying SN2 mechanisms .
Energy Profile
The energy diagram for an SN2 reaction typically shows a single transition state between reactants and products, consistent with its concerted mechanism. For gas-phase SN2 reactions, the energy profile often includes ion-dipole complexes before and after the central transition state, creating a characteristic potential energy surface with a central barrier flanked by energy wells .
Kinetic Isotope Effects
Kinetic isotope effects (KIEs) provide valuable mechanistic insights into SN2 reactions. For gas-phase SN2 reactions, slight inverse deuterium KIEs are typically observed at room temperature, resulting from a balance between normal rotational contributions and significant inverse vibrational contributions. Since vibrational contributions are temperature-dependent while rotational contributions remain relatively constant, KIEs for SN2 reactions show characteristic temperature dependence .
Table 2: Factors Affecting Kinetic Isotope Effects in SN2 Reactions
Factor | Effect on KIE | Temperature Dependence |
---|---|---|
Vibrational contribution | Inverse effect | Highly temperature-sensitive |
Rotational contribution | Normal effect | Temperature-independent |
Reaction barrier height | Determines tunneling importance | More important at low temperatures |
Reduced mass | Affects collision frequency | Slight temperature dependence |
Theoretical Models and Computational Studies
Transition State Theory
Transition state theory (TST) has been successfully applied to SN2 reactions, particularly those with significant energy barriers. This theoretical framework models the reaction rate based on the properties of the transition state relative to the reactants. For reactions with higher barriers (greater than approximately -4 kcal/mol), TST provides accurate predictions of rate constants and KIEs .
Ion-Molecule Collision Theory
For SN2 reactions with very low barriers, especially in the gas phase, ion-molecule collision theory offers a complementary framework. In these cases, the rate-limiting step becomes the collision frequency rather than overcoming an energy barrier. The capture rate constant for such reactions can be expressed using the Langevin-Gioumousis-Stevenson equation:
kₗ = 2πq(α/μ)½
Where q represents the ion charge, α is the polarizability of the neutral molecule, and μ is the reduced mass of the system .
Computational Methods
For reactions with intermediate barrier heights, the canonical unified statistical (CUS) theory, which incorporates both collision and transition state components, has proven effective in modeling rate constants and KIEs .
Applications in Organic Synthesis
- mass of a compound required to prepare a solution of known volume and concentration
- volume of solution required to dissolve a compound of known mass to a desired concentration
- concentration of a solution resulting from a known mass of compound in a specific volume