Spiro-NPB
CAS No.: 932739-76-9
Cat. No.: VC2672828
Molecular Formula: C57H38N2
Molecular Weight: 750.9 g/mol
* For research use only. Not for human or veterinary use.
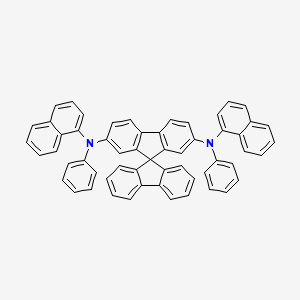
Specification
CAS No. | 932739-76-9 |
---|---|
Molecular Formula | C57H38N2 |
Molecular Weight | 750.9 g/mol |
IUPAC Name | 2-N',7-N'-dinaphthalen-1-yl-2-N',7-N'-diphenyl-9,9'-spirobi[fluorene]-2',7'-diamine |
Standard InChI | InChI=1S/C57H38N2/c1-3-21-41(22-4-1)58(55-31-15-19-39-17-7-9-25-45(39)55)43-33-35-49-50-36-34-44(59(42-23-5-2-6-24-42)56-32-16-20-40-18-8-10-26-46(40)56)38-54(50)57(53(49)37-43)51-29-13-11-27-47(51)48-28-12-14-30-52(48)57/h1-38H |
Standard InChI Key | ZDAWFMCVTXSZTC-UHFFFAOYSA-N |
SMILES | C1=CC=C(C=C1)N(C2=CC3=C(C=C2)C4=C(C35C6=CC=CC=C6C7=CC=CC=C57)C=C(C=C4)N(C8=CC=CC=C8)C9=CC=CC1=CC=CC=C19)C1=CC=CC2=CC=CC=C21 |
Canonical SMILES | C1=CC=C(C=C1)N(C2=CC3=C(C=C2)C4=C(C35C6=CC=CC=C6C7=CC=CC=C57)C=C(C=C4)N(C8=CC=CC=C8)C9=CC=CC1=CC=CC=C19)C1=CC=CC2=CC=CC=C21 |
Introduction
Chemical Structure and Properties
Spiro-NPB features a distinctive molecular structure characterized by a central spirobifluorene core with two diarylamine units attached at the 2 and 7 positions. The spirobifluorene component consists of two fluorene units connected through a spiro carbon atom, resulting in an orthogonal arrangement that contributes significantly to the compound's unique properties and performance characteristics .
The chemical formula of Spiro-NPB is C57H38N2 with a molecular weight of 750.9 g/mol. Its IUPAC name is 2-N',7-N'-dinaphthalen-1-yl-2-N',7-N'-diphenyl-9,9'-spirobi[fluorene]-2',7'-diamine, although it is also known by alternative chemical names including 2,7-Bis[N-(1-naphthyl)anilino]-9,9'-spirobi[9H-fluorene] and N,N'-Bis(naphthalen-1-yl)-N,N'-bis(phenyl)-9,9-spirobifluorene .
Table 1: Physical and Chemical Properties of Spiro-NPB
Spectroscopically, Spiro-NPB exhibits characteristic absorption and emission profiles that provide insight into its electronic structure. Its UV absorption maximum occurs at 380 nm when measured in tetrahydrofuran (THF) solution, while its photoluminescence peak is observed at 453 nm . A notable feature in its absorption spectrum is a smaller peak at approximately 308 nm, attributed to the π-π* transition of the fluorene units in the spiro core .
The compound demonstrates remarkable thermal stability with a decomposition temperature exceeding 360°C at 0.5% weight loss, making it suitable for applications requiring high-temperature processing or operation . This thermal resilience is largely attributed to the rigid spirobifluorene backbone, which constrains molecular motion and increases the energy required for decomposition, a critical factor for long-term device stability under operating conditions.
From an electronic perspective, Spiro-NPB possesses a highest occupied molecular orbital (HOMO) energy level of approximately 5.32 eV, which is strategically positioned to facilitate hole extraction from common anode materials while maintaining a suitable energy barrier to prevent excessive leakage current . Its triplet energy of 2.33-2.34 eV is crucial for its function in phosphorescent OLEDs, as it prevents back-energy transfer from the emissive dopants to the host material, thereby maximizing device efficiency .
Applications in Organic Electronics
Spiro-NPB finds its primary application as a hole transport material in organic electronic devices, with particular emphasis on OLEDs. The unique structural and electronic properties of Spiro-NPB make it especially suitable for this role, where efficient charge transport is critical for device performance .
In the architecture of an OLED, Spiro-NPB is typically positioned between the anode and the emissive layer. Its function is to facilitate the transport of holes from the anode (usually indium tin oxide) to the emissive layer where they combine with electrons to form excitons. These excitons subsequently decay radiatively to produce light, the fundamental process behind OLED operation.
The spirobifluorene core of Spiro-NPB contributes significantly to its effectiveness as a hole transport material. The orthogonal arrangement of the two fluorene units creates a three-dimensional molecular structure that inhibits close packing, reducing intermolecular interactions that could otherwise lead to crystallization and device degradation . This structural feature also contributes to the compound's high thermal stability, an important parameter for maintaining consistent performance during device operation.
Recent research has demonstrated the potential for spiro-type compounds in additional applications beyond traditional OLEDs. For example, a recent study published in January 2025 describes the development of a spiro-type self-assembled monolayer (SAM) for highly efficient and stable inverted perovskite solar cells . Although this specific research focused on a different spiro compound (4PA-spiro), it illustrates the broader potential of spiro-based materials in various organic electronic applications where efficient hole transport is required.
Performance in OLEDs
The performance of Spiro-NPB in OLED devices has been extensively studied, with particular attention to its comparison with other hole transport materials such as NPB (N,N′-bis(naphthalen-l-yl)-N,N′-bis(phenyl)-benzidine). Research findings indicate that OLEDs incorporating Spiro-NPB as the hole transport layer demonstrate several advantages over those using traditional materials .
These performance improvements include lower driving voltage, enhanced device power efficiency, and extended operational stability. The driving voltage reduction is particularly significant as it translates directly to lower power consumption, a critical parameter for commercial OLED applications . The enhanced stability, meanwhile, addresses one of the persistent challenges in OLED technology—device degradation over time—which is attributed to the suppressed crystallization process resulting from Spiro-NPB's rigid three-dimensional structure.
A specific study on red phosphorescence-based OLEDs provides quantitative performance data for devices utilizing Spiro-NPB and related compounds. In this research, devices employing a hole transport material similar to Spiro-NPB (identified as HTM 1B) achieved maximum current and power efficiencies of 16.16 cd/A and 11.17 lm/W, respectively, along with an external quantum efficiency of 13.64% . These values represent significant improvements over reference devices based on Spiro-NPB and NPB.
The superior performance of Spiro-NPB in OLEDs can be attributed to several factors:
-
Its appropriate HOMO energy level (approximately 5.32 eV) facilitates efficient hole injection from common anode materials .
-
The rigid spirobifluorene core provides enhanced thermal and morphological stability, preventing device degradation through crystallization .
-
Its suitable triplet energy (2.33-2.34 eV) prevents back-energy transfer in phosphorescent OLEDs, maximizing emission efficiency .
-
The three-dimensional structure reduces concentration quenching effects that can diminish luminescence efficiency.
Comparison with Other Hole Transport Materials
To fully appreciate the significance of Spiro-NPB, it is instructive to compare it with other prominent hole transport materials, particularly NPB, which has been widely used in OLED devices. The spirobifluorene core of Spiro-NPB represents the primary structural distinction from NPB, which features a biphenyl core instead.
Table 2: Comparison Between Spiro-NPB and Related Hole Transport Materials
Research indicates that Spiro-NPB offers improved device performance characteristics compared to conventional materials like NPB. The enhanced stability can be attributed to the suppressed crystallization process resulting from the rigid, three-dimensional structure of the spirobifluorene core . This structural feature inhibits molecular packing, thus preventing the formation of crystalline domains that can lead to device degradation.
Comparative studies between Spiro-NPB and novel hole transport materials (HTM 1A and HTM 1B) provide further insight into structure-property relationships. These materials share structural similarities with Spiro-NPB but feature different connecting motifs between the spirobifluorene core and the arylamine units . Notably, HTM 1B, which has a phenyl ring separating the central core and amine group, demonstrated superior device performance with an external quantum efficiency of 13.64%, exceeding that of Spiro-NPB-based devices .
The similar HOMO energy levels of Spiro-NPB and HTM 1A (approximately 5.32 eV) reflect their structural similarity, with both having amine derivatives directly attached to the central core. In contrast, HTM 1B exhibits a different HOMO level (5.54 eV) due to the intervening phenyl ring that alters the electronic density distribution . This comparison illustrates how subtle structural modifications can significantly impact the electronic properties and device performance of hole transport materials.
- mass of a compound required to prepare a solution of known volume and concentration
- volume of solution required to dissolve a compound of known mass to a desired concentration
- concentration of a solution resulting from a known mass of compound in a specific volume