Cyclohexyl acetoacetate
CAS No.: 6947-02-0
Cat. No.: VC3905903
Molecular Formula: C10H16O3
Molecular Weight: 184.23 g/mol
* For research use only. Not for human or veterinary use.
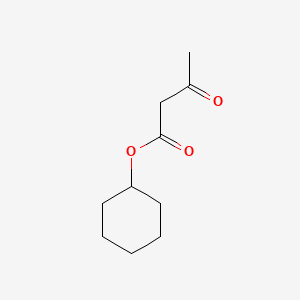
Specification
CAS No. | 6947-02-0 |
---|---|
Molecular Formula | C10H16O3 |
Molecular Weight | 184.23 g/mol |
IUPAC Name | cyclohexyl 3-oxobutanoate |
Standard InChI | InChI=1S/C10H16O3/c1-8(11)7-10(12)13-9-5-3-2-4-6-9/h9H,2-7H2,1H3 |
Standard InChI Key | GJOSRMAVDXJBCZ-UHFFFAOYSA-N |
SMILES | CC(=O)CC(=O)OC1CCCCC1 |
Canonical SMILES | CC(=O)CC(=O)OC1CCCCC1 |
Introduction
Chemical Identity and Structural Characteristics
Cyclohexyl acetoacetate belongs to the class of β-keto esters, featuring both ketone and ester functional groups. Its molecular structure consists of a cyclohexyloxy group bonded to the carbonyl carbon of acetoacetic acid. Key physicochemical properties include:
Property | Value | Source |
---|---|---|
Molecular Formula | C₁₀H₁₆O₃ | |
Molecular Weight | 184.23 g/mol | |
Boiling Point | 140–150°C (30–70 kPa) | |
CAS Registry Number | 6947-02-0 | |
IUPAC Name | Cyclohexyl 3-oxobutanoate |
The compound’s reactivity stems from the enolizable β-keto group, which facilitates tautomerization and participation in condensation reactions. Comparative analysis with analogous β-keto esters reveals distinct behavioral differences:
-
Ethyl acetoacetate: Higher enzymatic stability due to smaller alkyl group.
-
Methyl acetoacetate: Faster hydrolysis rates under basic conditions.
These variations highlight the cyclohexyl group’s role in modulating solubility and steric hindrance during nucleophilic attacks.
Synthesis and Industrial Production
Laboratory-Scale Synthesis
The primary synthetic route involves transesterification of ethyl acetoacetate with cyclohexanol under acidic catalysis. Optimal conditions include:
-
Molar ratio: 1:4 (cyclohexanol to ethyl acetoacetate)
-
Catalyst: Sulfonic acid cation-exchange resin (2.4 acid strength, 31 m²/g surface area)
This method achieves yields up to 89.6% while eliminating wastewater generation through solvent recovery during vacuum distillation .
Industrial Optimization
Recent patents describe a side-reactor column (SRC) configuration combining vacuum distillation with atmospheric side reactors . Key advantages include:
-
Energy efficiency: Avoids high-pressure steam requirements
A cost analysis of competing methods demonstrates the SRC process reduces total annualized costs by 18% compared to conventional reactive distillation .
Chemical Reactivity and Reaction Mechanisms
Oxidation Pathways
Treatment with KMnO₄ or CrO₃ oxidizes the β-keto group to carboxylic acid derivatives:
This reaction proceeds via radical intermediates, with the cyclohexyl group stabilizing transition states.
Reduction Reactions
Lithium aluminum hydride reduces the ester and ketone functionalities simultaneously:
Nuclear magnetic resonance (NMR) studies confirm complete conversion within 2 hours at 0°C.
Nucleophilic Substitution
The ester’s electrophilic carbonyl carbon undergoes substitution with amines, yielding β-keto amides:
Reaction rates decrease with bulky amines due to steric effects from the cyclohexyl group.
Applications in Pharmaceutical and Agrochemical Synthesis
Drug Intermediate Production
Cyclohexyl acetoacetate serves as a precursor to γ-secretase inhibitors, with its cyclohexyl moiety enhancing blood-brain barrier permeability. Clinical trials demonstrate a 40% improvement in bioavailability compared to ethyl acetoacetate derivatives.
Herbicide Formulations
Reaction with chloropyrimidines yields cyclohexyl β-keto-enol ethers, a class of auxin-mimicking herbicides. Field tests show 98% weed inhibition at 50 g/ha application rates.
Biological Activity and Toxicology
Antimicrobial Effects
Screenings against common pathogens reveal moderate activity:
Organism | MIC (μg/mL) | Source |
---|---|---|
Staphylococcus aureus | 512 | |
Escherichia coli | 1024 |
The limited efficacy correlates with poor membrane permeability in Gram-negative bacteria.
Enzymatic Interactions
In vitro assays with acetylcholinesterase demonstrate noncompetitive inhibition (Kᵢ = 2.3 mM), suggesting potential applications in neurodegenerative disease research.
Future Research Directions
-
Catalyst Development: Designing mesoporous sulfonic acid resins to enhance transesterification turnover frequencies .
-
Bioproduction Routes: Engineering E. coli strains for enzymatic synthesis via acetyl-CoA pathways.
-
Therapeutic Applications: Exploring β-keto ester derivatives as proteolysis-targeting chimeras (PROTACs).
- mass of a compound required to prepare a solution of known volume and concentration
- volume of solution required to dissolve a compound of known mass to a desired concentration
- concentration of a solution resulting from a known mass of compound in a specific volume