LNA-Adenosine
CAS No.: 206055-70-1
Cat. No.: VC0533407
Molecular Formula: C11H13N5O4
Molecular Weight: 279.26
Purity: >98% (or refer to the Certificate of Analysis)
* For research use only. Not for human or veterinary use.
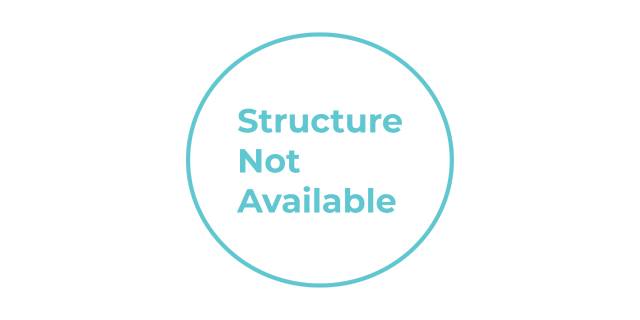
Specification
CAS No. | 206055-70-1 |
---|---|
Molecular Formula | C11H13N5O4 |
Molecular Weight | 279.26 |
IUPAC Name | (1S,3R,4R,7S)-3-(6-aminopurin-9-yl)-1-(hydroxymethyl)-2,5-dioxabicyclo[2.2.1]heptan-7-ol |
Standard InChI | InChI=1S/C11H13N5O4/c12-8-5-9(14-3-13-8)16(4-15-5)10-6-7(18)11(1-17,20-10)2-19-6/h3-4,6-7,10,17-18H,1-2H2,(H2,12,13,14)/t6-,7+,10-,11+/m1/s1 |
Standard InChI Key | GHKDRNDFVCEETA-KYAGDKKUSA-N |
SMILES | C1C2(C(C(O1)C(O2)N3C=NC4=C(N=CN=C43)N)O)CO |
Appearance | Solid powder |
Introduction
Structure and Chemical Properties of LNA-Adenosine
Structural Characteristics
LNA-Adenosine (often abbreviated as LNA-A) features the distinctive structural element that defines all locked nucleic acids: a methylene bridge connecting the 2' oxygen and 4' carbon atoms of the ribose sugar. This bridge effectively "locks" the ribose in the 3'-endo (North) conformation, which naturally occurs in A-form RNA duplexes . The rigid conformation imposed by this bridging significantly affects the molecule's binding properties and stability. Unlike standard adenosine, which can switch between different conformational states, LNA-Adenosine remains fixed in this optimal binding configuration, enhancing its interaction with complementary nucleic acids.
Physical and Chemical Properties
LNA-Adenosine exhibits remarkable thermal stability when incorporated into oligonucleotide structures. The constrained conformation pre-arranges the nucleobase for optimal stacking, significantly increasing enthalpy gain upon duplex formation while simultaneously reducing entropy loss . This translates to substantially higher melting temperatures (Tm) compared to both DNA and RNA counterparts containing standard adenosine.
When incorporated into oligonucleotides, LNA-Adenosine creates local reorganization of the phosphate backbone. This reorganization often extends to neighboring nucleotides, steering their conformations toward the A-form helix structure . The locked conformation also contributes to enhanced resistance against nuclease degradation, making LNA-Adenosine-containing oligonucleotides particularly valuable for in vivo applications where stability is crucial.
Synthesis of LNA-Adenosine
Historical Development
The development of LNA-Adenosine traces back to the pioneering work on locked nucleic acids. Obika et al. first chemically synthesized LNA in 1997, with Jesper Wengel's group independently developing similar compounds in 1998 . These initial breakthroughs built upon the foundational work of Zamecnick and Stephenson, who in 1978 established the potential of oligonucleotides as agents for controlling gene expression . Since these early developments, synthetic approaches have been refined to produce high-quality LNA-Adenosine with improved yields and purity.
Synthetic Strategies
Two primary approaches have emerged for synthesizing LNA-Adenosine and other LNA nucleosides: linear and convergent strategies. Each offers distinct advantages depending on the specific requirements of the synthesis.
Linear Strategy
The linear strategy, initially detailed by Obika et al., typically begins with a readily available RNA nucleoside such as uridine . For LNA-Adenosine synthesis, this approach would require additional steps to exchange the base moiety from uracil to adenine. The linear strategy involves sequential modifications of the starting nucleoside to introduce the 2'-O,4'-C-methylene bridge while preserving the desired stereochemistry. This approach offers certain advantages for scale-up and specific modifications but may involve more synthetic steps.
Convergent Strategy
The convergent strategy requires synthesizing a sugar intermediate that serves as a glycosyl donor for coupling with nucleobases. Typically, D-glucose serves as the starting material to produce the sugar intermediate, which is subsequently reacted with adenine using a modified Vorbrüggen procedure that allows for stereoselective coupling . This approach allows for more efficient synthesis of various LNA nucleosides by changing only the nucleobase component in the coupling step.
Synthesis Challenges and Byproducts
During the chemical synthesis of LNA-Adenosine, researchers have observed the formation of N7-LNA-adenosine (7AL) as a minor product . This isomeric form results from glycosylation at the N7 position of adenine rather than the canonical N9 position. The presence of such byproducts highlights the complexity of the stereoselective synthesis process and necessitates careful purification protocols to ensure product homogeneity.
Recent advances in synthetic methodologies have focused on improving yield and stereoselectivity while reducing the formation of such byproducts. These improvements have made LNA-Adenosine more accessible for research and commercial applications.
Incorporation into Oligonucleotides
Chemical Oligonucleotide Synthesis
LNA-Adenosine can be incorporated into oligonucleotides through standard solid-phase synthesis techniques using phosphoramidite chemistry. The process involves converting LNA-Adenosine to its corresponding phosphoramidite derivative, which can then be used in automated DNA synthesizers . This compatibility with established synthetic protocols has facilitated the widespread adoption of LNA-Adenosine in various research applications.
When incorporated into oligonucleotides, LNA-Adenosine can be combined with other nucleotide types, including standard DNA/RNA nucleotides or other modified nucleosides. This flexibility allows researchers to fine-tune the properties of the resulting oligonucleotides for specific applications.
Enzymatic Incorporation
Beyond chemical synthesis, certain DNA and RNA polymerases can incorporate LNA nucleotides, including LNA-Adenosine, into growing nucleic acid chains . This enzymatic approach offers an alternative method for creating LNA-containing oligonucleotides, though it typically requires careful optimization of reaction conditions. The promiscuity of select polymerases enables the incorporation of LNA-Adenosine triphosphate into nucleic acid structures through enzymatic synthesis, expanding the toolbox for creating functional LNA-containing molecules.
Functional Properties and Applications
Enhanced Hybridization Properties
LNA-Adenosine significantly contributes to the exceptional hybridization properties of LNA-containing oligonucleotides. When incorporated into DNA or RNA strands, LNA-Adenosine enhances binding to complementary sequences through several mechanisms:
-
Increased thermal stability of duplexes, with each LNA-Adenosine potentially raising the melting temperature by 1-8°C depending on sequence context
-
Improved base stacking interactions
-
Favorable pre-organization of the nucleotide in a conformation conducive to duplex formation
These properties make LNA-Adenosine-containing oligonucleotides particularly valuable for applications requiring high-affinity binding to target sequences.
Nuclease Resistance
The structural constraints imposed by the locked conformation render LNA-Adenosine significantly more resistant to nuclease degradation compared to standard adenosine. When incorporated into oligonucleotides, LNA-Adenosine contributes to enhanced stability against both exonucleases and endonucleases . This increased stability is particularly beneficial for in vivo applications where nuclease degradation represents a major challenge for nucleic acid-based therapeutics.
The combination of heightened nuclease resistance and preserved water solubility makes LNA-Adenosine-containing oligonucleotides highly suitable for therapeutic applications without requiring extensive modification of experimental protocols .
Applications in Research and Therapeutics
LNA-Adenosine has found applications across numerous fields of molecular biology and medicine:
Antisense Technology
LNA-Adenosine-containing oligonucleotides demonstrate superior antisense inhibition properties, particularly against small RNA targets . The enhanced binding affinity and specificity enable effective target recognition at lower concentrations compared to conventional antisense agents. Additionally, the strand invasion properties conferred by LNA modifications, including LNA-Adenosine, make these oligonucleotides particularly effective for targeting structured RNA regions that might be inaccessible to standard oligonucleotides.
Diagnostic Applications
The exceptional sequence specificity of LNA-Adenosine-containing probes enables detection of single nucleotide mismatches, making them valuable tools for genotyping and mutation detection . These probes can discriminate between highly similar sequences, including members of miRNA families that differ by only a single nucleotide. This discriminatory power is essential for applications requiring precise target identification.
Therapeutic Nucleic Acids
LNA-Adenosine contributes to the development of therapeutic oligonucleotides by:
-
Enhancing target binding affinity, allowing for shorter therapeutic sequences
-
Improving nuclease resistance for extended in vivo half-life
-
Enabling more efficient cellular uptake in some contexts
-
Contributing to reduced off-target effects through enhanced specificity
These properties make LNA-Adenosine a valuable component in the design of therapeutic oligonucleotides targeting various disease pathways.
Comparative Analysis
Advantages and Limitations
LNA-Adenosine offers several distinct advantages:
-
Significantly enhanced target binding affinity
-
Superior nuclease resistance
-
Excellent discrimination between closely related sequences
-
Compatibility with standard nucleic acid handling protocols
-
Ability to function in various biological environments
-
Higher synthesis costs compared to standard nucleosides
-
Potential formation of byproducts like N7-LNA-adenosine during synthesis
-
Possible altered biodistribution compared to standard oligonucleotides
-
Limited structural flexibility which may be disadvantageous for certain applications
Research Developments and Future Perspectives
Recent research has continued to expand the applications and understanding of LNA-Adenosine. The experimental and computational investigations into RNA duplexes containing LNA have provided deeper insights into the structural impacts of LNA incorporation . These studies enhance our understanding of how LNA-Adenosine affects nucleic acid structures and interactions.
The continued refinement of synthesis methods for LNA-Adenosine and related compounds suggests an expanding role for these modified nucleosides in future applications. Current trends indicate growing interest in combinatorial approaches that incorporate LNA-Adenosine alongside other modifications to create oligonucleotides with precisely tailored properties for specific therapeutic and diagnostic applications.
- mass of a compound required to prepare a solution of known volume and concentration
- volume of solution required to dissolve a compound of known mass to a desired concentration
- concentration of a solution resulting from a known mass of compound in a specific volume