Lithium acrylate
CAS No.: 13270-28-5
Cat. No.: VC20976767
Molecular Formula: C3H3LiO2
Molecular Weight: 78 g/mol
* For research use only. Not for human or veterinary use.
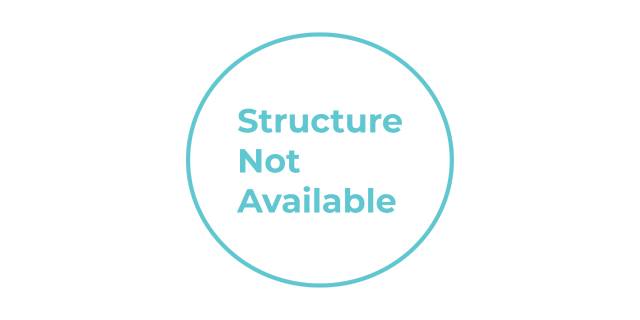
Specification
CAS No. | 13270-28-5 |
---|---|
Molecular Formula | C3H3LiO2 |
Molecular Weight | 78 g/mol |
IUPAC Name | lithium;prop-2-enoate |
Standard InChI | InChI=1S/C3H4O2.Li/c1-2-3(4)5;/h2H,1H2,(H,4,5);/q;+1/p-1 |
Standard InChI Key | XSAOIFHNXYIRGG-UHFFFAOYSA-M |
Isomeric SMILES | [Li+].C=CC(=O)[O-] |
SMILES | [Li+].C=CC(=O)[O-] |
Canonical SMILES | [Li+].C=CC(=O)[O-] |
Introduction
Fundamental Properties and Characteristics
Chemical Identity and Structure
Lithium acrylate is an organolithium compound with the molecular formula C3H3LiO2 and a molecular weight of 78.0 g/mol. It consists of an acrylate anion (CH2=CHCOO-) paired with a lithium cation (Li+). The compound's IUPAC name is lithium;prop-2-enoate, and it is identified by the InChI Key XSAOIFHNXYIRGG-UHFFFAOYSA-M. Its canonical SMILES notation is represented as [Li+].C=CC(=O)[O-].
Physical Properties
Lithium acrylate typically appears as a white crystalline solid that exhibits solubility in water and polar organic solvents. This solubility profile makes it suitable for various processing methods in laboratory and industrial applications. The compound's physical characteristics are influenced by its ionic nature, which contributes to its stability and reactivity in different environments.
Property | Value |
---|---|
Molecular Formula | C3H3LiO2 |
Molecular Weight | 78.0 g/mol |
CAS Number | 13270-28-5 |
Physical State | White crystalline solid |
Solubility | Soluble in water and polar organic solvents |
IUPAC Name | lithium;prop-2-enoate |
InChI Key | XSAOIFHNXYIRGG-UHFFFAOYSA-M |
Synthesis Methodologies
Standard Preparation Methods
The primary method for synthesizing lithium acrylate involves the neutralization reaction between acrylic acid and lithium hydroxide in an aqueous medium. This reaction can be represented by the following equation:
CH2=CHCOOH + LiOH → CH2=CHCOOLi + H2O
The synthesis is typically conducted under controlled conditions to ensure high purity of the final product. Temperature, pH, and reactant concentrations are carefully monitored to optimize yield and product quality.
Advanced Synthesis Approaches
Recent advancements in synthesis methodologies have focused on improving the efficiency and scalability of lithium acrylate production. These approaches include:
-
Utilization of alternative lithium sources such as lithium carbonate
-
Implementation of continuous flow processes for industrial-scale production
-
Development of green chemistry approaches to minimize environmental impact
-
Employment of specialized catalysts to enhance reaction rates and selectivity
Purification Techniques
After synthesis, various purification methods can be employed to obtain high-purity lithium acrylate:
-
Recrystallization in appropriate solvents
-
Vacuum drying procedures (typically at 60°C for 24 hours)
-
Characterization via Fourier-transform infrared spectroscopy (FTIR) and X-ray diffraction (XRD)
-
Analytical validation using techniques such as nuclear magnetic resonance (NMR) spectroscopy
Polymerization Behavior
Radical Polymerization Mechanisms
Lithium acrylate undergoes radical polymerization to form poly(lithium acrylate), with the process typically initiated by compounds such as azobisisobutyronitrile (AIBN) or benzoyl peroxide. The polymerization is generally conducted under controlled temperature conditions (70-80°C) and an inert atmosphere (nitrogen). This process results in high-molecular-weight polymers with molecular weights exceeding 500,000 g/mol under optimized conditions.
Copolymerization Characteristics
The compound readily copolymerizes with various monomers, including alkyl acrylates and styrene, allowing for the tailoring of polymer properties for specific applications. The reactivity ratios in these copolymerization reactions significantly influence the final composition and properties of the resulting materials.
Polymerization Parameter | Typical Conditions/Values |
---|---|
Polymerization Method | Radical polymerization |
Common Initiators | AIBN, benzoyl peroxide |
Optimal Temperature Range | 70-80°C (under nitrogen) |
Secondary Reactions | Backbiting, β-scission (minimized under controlled conditions) |
Resulting Polymer Properties | High ionic conductivity, thermal stability |
Thermal Behavior During Polymerization
Thermogravimetric analysis (TGA) studies have shown that poly(lithium acrylate) begins to undergo thermal decomposition at approximately 200°C, with complete decomposition occurring by 400°C. The decomposition process involves β-scission and depropagation reactions, resulting in the formation of low molecular weight fragments and traces of lithium carbonate.
Electrolyte System | Ionic Conductivity (S cm^-1) | Temperature (°C) | Reference Features |
---|---|---|---|
UV-cured polyurethane acrylate with lithium salts | 1.00 × 10^-4 | 25 | Ambient temperature operation |
PEO/PMA blends with lithium acrylate | Variable based on composition | 25-80 | Suppressed crystallinity |
Crosslinked systems with diacrylates | Up to 10^-4 | 25 | Enhanced mechanical properties |
Biological and Antimicrobial Properties
Antimicrobial Activity Profiles
Recent research has revealed interesting antimicrobial properties of lithium acrylate when incorporated into copolymer matrices. Studies investigating the synthesis of copolymer films containing lithium acrylate have demonstrated significant bactericidal activity against several microbial strains, particularly Staphylococcus aureus.
Microorganism | Inhibition Zone (mm) |
---|---|
Staphylococcus aureus | 15 |
Escherichia coli | 12 |
Candida albicans | 10 |
Chemical Reactivity Profiles
Ion-Exchange Reactions
In lithium-ion battery applications, lithium acrylate-based binders participate in reversible H+/Li+ exchange reactions at electrode interfaces. This mechanism contributes to improved lithium-ion transport, reduced internal resistance, and enhanced cycle stability, with some systems demonstrating greater than 91% capacity retention after 1,400 cycles.
Crosslinking Reactions
Lithium acrylate readily participates in UV-initiated crosslinking reactions with diacrylates, such as 1,6-hexanediol diacrylate. These reactions form network structures that serve as effective electrolyte matrices with tunable mechanical and electrochemical properties.
Stability in Aqueous Environments
Lithium acrylate emulsions (with particle sizes ranging from 50-200 nm) demonstrate good stability in aqueous environments due to electrostatic repulsion between carboxylate groups. Hydrolysis is minimal under neutral pH conditions, though acidic environments can promote the regeneration of acrylic acid.
Comparative Analysis with Related Compounds
Structural and Functional Comparisons
Lithium acrylate belongs to a family of alkali metal acrylates, each with distinct properties and applications. The table below presents a comparative analysis:
Compound | Formula | Key Differences from Lithium Acrylate |
---|---|---|
Sodium Acrylate | C3H3NaO2 | Larger ionic radius, lower specific conductivity for Li+ transport applications |
Potassium Acrylate | C3H3KO2 | Significantly larger cation size, different polymer network properties |
Lithium Methacrylate | C4H5LiO2 | Contains methyl group, altered polymerization kinetics and mechanical properties |
Performance Differentiation in Applications
When compared to other acrylate salts, lithium acrylate demonstrates superior performance in several application areas:
-
Enhanced ionic conductivity specifically for lithium-ion transport
-
Better compatibility with lithium-ion battery components
-
Unique polymer network characteristics when polymerized
-
Specific thermal decomposition patterns beneficial for certain applications
Future Research Directions and Challenges
Emerging Research Trends
Current research on lithium acrylate is advancing in several promising directions:
-
Development of green synthesis methods with reduced environmental impact
-
Exploration of novel copolymer systems with enhanced functional properties
-
Investigation of structure-property relationships in lithium acrylate-based materials
-
Integration with nanomaterials for advanced composite structures
Technical Challenges
Despite its promising applications, several challenges remain in lithium acrylate research and development:
-
Scaling up production processes while maintaining consistent product quality
-
Improving the stability of lithium acrylate-based materials under extreme environmental conditions
-
Enhancing the mechanical properties of resulting polymers for structural applications
-
Addressing cost considerations for wider commercial adoption
- mass of a compound required to prepare a solution of known volume and concentration
- volume of solution required to dissolve a compound of known mass to a desired concentration
- concentration of a solution resulting from a known mass of compound in a specific volume